High-Energy Astrofysica en Active Galaxies
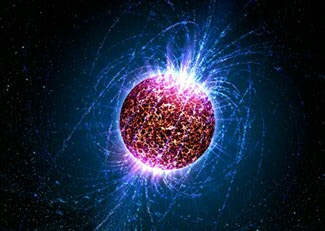
Aan het einde van het leven van een zeer massieve ster (>~ 8 zonsmassa's) kan de zwaartekracht de protonen en elektronen in de kern van de ster dwingen zich te combineren tot neutronen, waardoor een neutronenster (~1-2 zonsmassa's, straal van 10-15 km) wordt gevormd. De rest van de ster wordt in dit proces weggeblazen, ook wel een Supernova Type II genoemd. Verdere instorting wordt voorkomen door de afstoting tussen de neutronen als gevolg van het Pauli uitsluitingsprincipe (ook bekend als de druk van een degeneraat neutronengas).
Neutronensterren
Neutronensterren hebben sterke magnetische velden en zijn snelle rotators. Ook van pulsars wordt aangenomen dat het neutronensterren zijn die zo snel roteren dat ze een stralingsbundel uitzenden langs hun magnetische as, die niet noodzakelijkerwijs is uitgelijnd met hun rotatieas. Net als een vuurtoren kan deze straal, wanneer hij naar de aarde wijst, worden opgepikt als een pulsar.
Zwarte gaten
Bij nog massievere sterren kan de kern zo massief zijn dat geen enkel bekend mechanisme verdere instorting tot een singulariteit kan voorkomen: er ontstaat een zwart gat. Deze objecten hebben de sterkste zwaartekrachtvelden in het heelal. Zelfs licht kan niet ontsnappen als het te dicht bij de singulariteit is. Kwantummechanische effecten zorgen er echter wel voor dat zwarte gaten de zogenaamde Hawkingstraling uitzenden en uiteindelijk verdampen, lang nadat de laatste sterren in het heelal zijn gestorven. Hun sterke zwaartekracht trekt gas aan van nabijgelegen sterren, dat een schijf vormt wanneer het is geaccretiseerd, en zo sterk opwarmt dat het röntgenstraling kan uitzenden.
We kunnen meer te weten komen over de dynamica in de omgeving van het zwarte gat door de emissie in de röntgenstralingbanden te bestuderen. Er zijn ook sterke redenen om aan te nemen dat de centra van sterrenstelsels vaak superzware zwarte gaten herbergen, en sommige laten hun activiteit zelfs zien als AGN's en quasars. Zowel neutronensterren als zwarte gaten vertonen ook afdrukken van de kromming van de ruimtetijd zoals voorspeld door de Algemene Relativiteit, wat sterke implicaties heeft voor de astrofysica en de kosmologie in het algemeen.
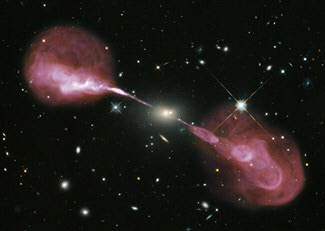
Belangrijke vragen
Onderzoek bij het Kapteyn Instituut richt zich op de fysica van accretie op neutronensterren en zwarte gaten met lage massa, de effecten van extreem sterke zwaartekrachtvelden en de interne samenstelling van neutronensterren.
In het bijzonder worden de volgende vragen onderzocht: Wat zijn de eigenschappen van materie onder de extreme omstandigheden die heersen in het inwendige van een neutronenster? En wat zijn de waargenomen kenmerken van zwarte gaten? Wat zijn de fysische en geometrische eigenschappen van de accretiestroom in deze systemen en hoe houdt accretie verband met de relativistische jets die op radiogolven worden waargenomen? Hoe gedragen nabije deeltjes en straling zich, en kunnen we de buitengewone voorspellingen van algemene relativiteit voor de eigenschappen van gekromde ruimtetijd in de buurt van deze objecten observationeel verifiëren?
Kapteyn-medewerkers werken aan de symbiose tussen actieve galactische kernen (AGN's) en sterrenhopen in krachtige AGN's, eenwording van AGN's en het voorkomen van ultrafijne AGN's in diepe velden. Daarnaast worden de samenstelling en kinematica van de sterren en de ISM in AGN-gastheerstelsels en de eigenschappen van AGN-gestuurde radiobronnen, inclusief restanten, zowel op zichzelf als in hun IGM-interactie bestudeerd.
Projecten
Enkele projecten en programma's waarbij het instituut betrokken is, zijn multi-golflengte studies van AGN met LOFAR, Herschel, Spitzer, en studies van neutronensterren en zwarte gaten met XMM-Newton en NICER.
Toekomstig onderzoek
Toekomstige richtingen van hoogenergetische astrofysica en AGN-onderzoek aan het Kapteyn Instituut richten zich op de voorgestelde röntgentelescoop ATHENA samen met SRON, en het gebruik van LOFAR/SKA in AGN-studies. Verder het gebruik van ALMA, SPICA/SAFARI bij AGN-studies en het gebruik van APERTIF (ARTS) bij het bestuderen van pulsars, roterende radiotransits en snelle radio-uitbarstingen.
Wetenschappelijke staf
Wetenschappers die op dit gebied werkzaam zijn, zijn: Barthel, Caputi, Mendez, Morganti, Wang.
Kosmische stralen
Kosmische stralen zijn subatomaire deeltjes die versneld worden in explosieve kosmische gebeurtenissen of objecten die aangedreven worden door sterke zwaartekracht. Meer dan een eeuw na hun ontdekking moeten de bronnen van kosmische straling nog steeds ondubbelzinnig worden geïdentificeerd, omdat ze zich niet in rechte banen voortbewegen. Wanneer kosmische stralen echter botsen met materie en straling, produceren ze gammastralen met zeer hoge energie (VHE) die rechtstreeks terugverwijzen naar hun verre geboorteplaatsen.
Deze gammastralen bevatten de sleutel tot enkele van de meest diepgaande vragen over ons heelal en bieden een overzicht van de objecten waar de extremen van materie heersen, zoals zwarte gaten, neutronensterren of hun fusiegebeurtenissen die zwaartekrachtgolven produceren. VHE-gammastralen beloven ook de ware aard van donkere materie te onthullen.
Onderzoek kosmische stralen
Het onderzoek aan het Kapteyn Instituut richt zich op het hoogenergetische heelal zoals gezien in kosmische en gammastralen, zowel vanuit experimenteel als fenomenologisch oogpunt. Kapteyn-medewerkers zijn betrokken bij de detectie van kosmische straling met de Alpha Magnetic Spectrometer in de ruimte en bij de detectie van gammastraling met het High Energy Stereoscopic System (HESS)-experiment en de aankomende Cherenkov Telescope Array (CTA).
Wetenschappelijke staf kosmische stralen
Wetenschappers op dit gebied: Manuela Vecchi.
Laatst gewijzigd: | 22 juli 2025 11:38 |