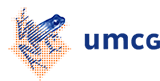
3. Molecular mechanisms underlying the development of acute myeloid leukemia
Our studies can be divided in several subprojects:
1. Building retro/lentiviral transduction models that recapitulate human leukemias
2. Gene expression profiling of leukemic stem cell-enriched populations
3. Role of epigenetics in (leukemic) stem cells
4. Screening for novel leukemic stem cell markers
5. Evaluation of the efficacy of available drugs, small-molecule inhibitors and novel approaches to target leukemic stem cells (simvastatin, dasatinib, Trail, Rac inhibitors)
6. The role of autophagy
Acute myeloid leukemia (AML) consists of a heterogeneous population of malignant cells that appears to be hierarchically structured, just as the normal hematopoietic system (Figure 1). This heterogeneous population of AML cells contains only a limited number of primitive cells that are characterized by the immunophenotypic markers CD34+/CD38- and long-term engraftment potential in NOD-SCID mice. The leukemic stem cells (LSCs) have properties in common with normal HSCs, especially the capacity of stem cell self-renewal, but differ with regard to the differentiation program, which is often impaired. Apparently, the transforming events in HSCs or Multipotent Progenitor Cells (MPPs) trigger a genetic program that deregulates the differentiation program at several levels and enhances the self-renewal potential of immature stem and/or progenitor cells. At the molecular level this is reflected by the sustained activation of a number of proteins instead of the normal pattern of transient phosphorylation and de-phosphorylation. Recent studies in our lab have shown that the sustained activation of the transcription factor STAT5 in cord blood stem cells triggers the self-renewal potential of HSCs and strongly influences the interaction of HSCs with stromal cells, but limits differentiation along the myeloid lineage. This transcription factor is of interest in AML since a high number of AML cases demonstrate sustained STAT5 tyrosine phosphorylation due to mutations in cytokine receptors (Flt3, c-Kit), several chromosomal translocation products such as Tel/Jak2 and Tel/Abl, or due to autocrine produced growth factors. Apparently, this transcription factor has great impact on the genetic program that controls relevant properties of HSCs. The aim of this research line is to elucidate mechanisms by which several cellular defects in AML that converge at the level of STAT5 activation can control self-renewal and hampered myelopoiesis of the developing leukemic clone. We have optimized culture conditions and lentiviral transdution procedures for AML cells that allow gene-function analysis in pramry AML cells from patients. Besides STAT5, we have generated retro/lentiviral models for K-RAS, BMI1, Rac1/2, FLT3-ITD, MLL-AF9, MLL-ENL, NPM, p16, AMl1-ETO and BCR-ABL (Figure 2).
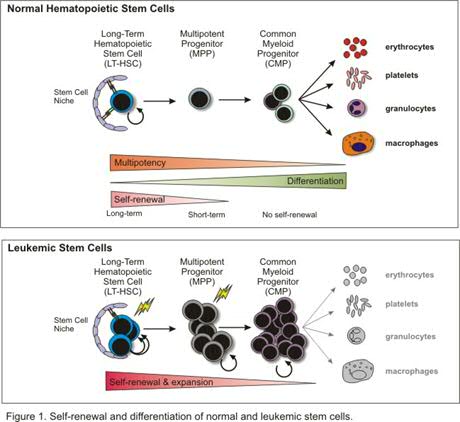
Leukemic transformation has often been described as a multi-step process, in which an initial mutation results in enhanced proliferation, which in conjunction with mutations in the differentiation and apoptotic program, synergize in the leukemic event. Although our preliminary data indicate that a persistent activation of STAT5 in human CB CD34+ cells results in enhanced self-renewal on MS5 stromal cells, it is currently unclear whether this persistent activation of STAT5 is also sufficient to induce a leukemia-like phenotype in NOD-SCID mice. It is plausible that, as is the case for e.g. AML1-ETO, one single hit is not sufficient to induce a full leukemic phenotype in vivo. We are currently pursuing to build a multi-step leukemic transformation model in which various combinations of oncogenes (eg BCR-ABL with BMI1, KRAS with BMI1) are introduced into human stem and progenitor cells to deepen our insights into the development of leukemia.
Furthermore, we have begun to address the question: where do leukemic stem cells reside in the bone marrow? (Figure 3). Do leukemic stem cells also depend on a niche as normal stem cells do? Or do they have a different relationship with the niche, but still need it in order to orchestrate self-renewal divisions? While low oxygen levels are thought to be important to regulate stem cell quiescence in the in the endosteal niche, do leukemic stem cells require similar hypoxic niches to maintain their quiescence?
Also, we have begun to analyse gene expression profiles in leukemic stem cell enriched CD34+ populations in comparison to leukemic stem cell-depleted CD34- populations, in order to gain further insight into the process of leukemic transformation.
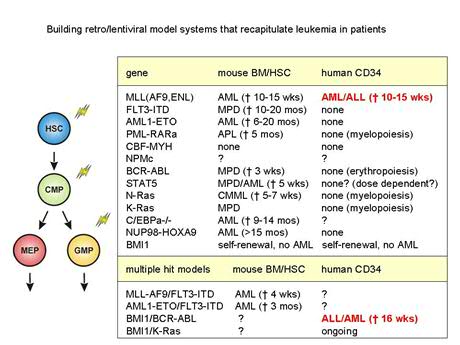
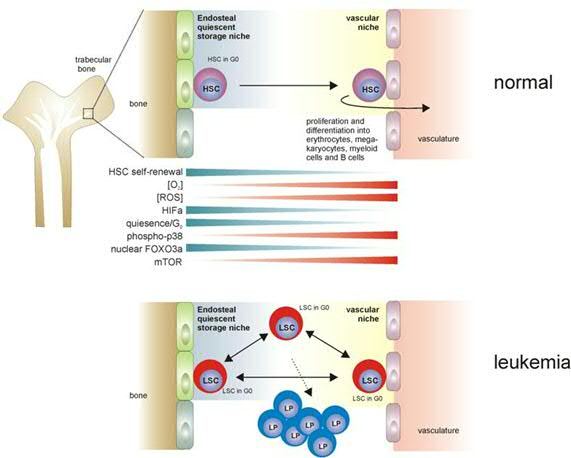
Even though the NSG xenograft mouse model is often considered to be the gold standard for human hematoppoiesis/leukemia studies, these mice are rather B-cell lymphoid-biased and many patient samples either do not engraft at all or fail te repopulate secondary reciepients due to loss of stemness. Most likely this is related to the murine bone marrow niche, which does not provide the essential human growth factors, cytokines and adhesion factors that are required for efficient maintenance of stemness. Therefore, we have begun to to generate human niche in xenograft mouse models in order to better recapitulate human stem cell biology and leukemogenesis in vivo. By making use of primary Mesenchymal Stem Cells (MSCs) that we implant subcutaenously in NSG mice we have been able to recreate a human niche in the mouse. Thus, we have established a mouse leukemia clinic in which all important subgroups are represented. These models have proven to be very useful to study molecular mechanisms underlying leukemia development and are also excellent platforms to evaluate novel therapeutic approaches and drugs. For further info, please see:
Establishing human leukemia xenograft mouse models by implanting human bone marrow-like scaffold-based niches. Antonelli A, Noort WA, Jaques J, de Boer B, de Jong-Korlaar R, Brouwers-Vos AZ, Lubbers-Aalders L, van Velzen JF, Bloem AC, Yuan H, de Bruijn JD, Ossenkoppele GJ, Martens AC, Vellenga E, Groen RW, Schuringa JJ. Blood. 2016 Dec 22;128(25):2949-2959. doi: 10.1182/blood-2016-05-719021. Epub 2016 Oct 12.
Genetically engineered mesenchymal stromal cells produce IL-3 and TPO to further improve human scaffold-based xenograft models. Carretta M, de Boer B, Jaques J, Antonelli A, Horton SJ, Yuan H, de Bruijn JD, Groen RWJ, Vellenga E, Schuringa JJ. Exp Hematol. 2017 Jul;51:36-46. doi:0.1016/j.exphem.2017.04.008. Epub 2017 Apr 26.
Modeling BCR-ABL and MLL-AF9 leukemia in a human bone marrow-like scaffold-based xenograft model. Sontakke P, Carretta M, Jaques J, Brouwers-Vos AZ, Lubbers-Aalders L, Yuan H, de Bruijn JD, Martens AC, Vellenga E, Groen RW, Schuringa JJ. Leukemia. 2016 Oct;30(10):2064-2073. doi: 10.1038/leu.2016.108. Epub 2016 Apr 29.
For more information on these projects, please contact J.J.Schuringa: j.j.schuringa@umcg.nl
Last modified: | 21 September 2017 1.51 p.m. |