Watching energy transport through biomimetic nanotubes
Simple Science Summary
Plants and some bacteria catch light with special light-harvesting antennae, and use the energy. These systems are very efficient, so scientists are curious to find out how they work. But the systems are also very complex. Now, scientist from the University of Groningen have created a simple artificial version of such an antenna and studied how it catches light and transports the energy. The results could be used in making molecular ‘wires’ for the transport of energy.
Scientists from the University of Groningen and the University of Würzburg (Germany) have investigated a simple biomimetic light-harvesting system using advanced spectroscopy combined with a microfluidic platform. The double-walled nanotubes work very efficiently at low light intensities, while they are able to get rid of excess energy at high intensities. These properties are useful in the design of novel materials for the harvesting and transport of photon energy. The results were published in the journal Nature Communications on 10 October.
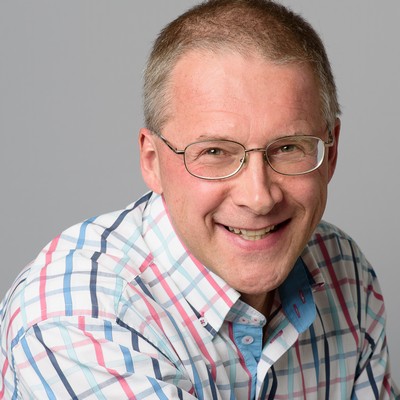
The remarkable ability of natural photosynthetic complexes to efficiently harness sunlight – even in dark environments – has sparked widespread interest in deciphering their functionality. Understanding energy transport on the nanoscale is key for a range of potential applications in the field of (opto)electronics. The overwhelming complexity of natural photosynthetic systems, consisting of many hierarchically arranged sub-units, led scientists to turn their attention to biomimetic analogs, which are structured like their natural counterparts but can be more easily controlled.
Ligh-harvesting molecules

The Optical Condensed Matter Science group and the Theory of Condensed Matter group (both at the Zernike Institute for Advanced Materials, University of Groningen) have joined forces with colleagues from the University of Würzburg (Germany) to gain a comprehensive picture of energy transport in an artificial light-harvesting complex. They used a new spectroscopic lab-on-a-chip approach, which combines advanced time-resolved multidimensional spectroscopy, microfluidics and extensive theoretical modelling.
The scientists investigated an artificial light-harvesting device, inspired by the multi-walled tubular antenna network of photosynthetic bacteria found in nature. The biomimetic device consists of nanotubes made out of light-harvesting molecules, self-assembled into a double-walled nanotube. ‘However, even this system is rather complex,’ explains Maxim Pshenichnikov, professor of ultrafast spectroscopy at the University of Groningen. His group devised a microfluidic system, in which the outer wall of the tube can be selectively dissolved and, thus, switched off. ‘This is not stable, but in the flow system, it can be studied.’ In this way, the scientists could study both the inner tube and the complete system.

At low light intensity, the system absorbs photons in both walls, creating excitations or excitons. ‘Due to the different sizes of the walls, they absorb photons of different wavelengths,’ Pshenichnikov explains. ‘This increases the efficiency.’ At high light intensity, a large number of photons is absorbed, creating a huge number of excitons. ‘We observed that, when two excitons meet, one of them actually ceases to exist.’ This effect acts as a kind of safety valve, as high numbers of excitons could damage the nanotubes.
Molecular materials
Thus, the scientists also demonstrated that the double-walled molecular nanotube is capable of adapting to changing illumination conditions. They mimic the essential functional elements of nature’s design toolbox at low light conditions by acting as highly sensitive antennas but get rid of excess energy at high intensities when there is too much light – a situation that would not normally occur in nature. Both t hese properties pave the way to better control of the transport of energy through complex molecular materials.
This paper was selected as 'Editors' Highlight' by the Nature editorial team.
Reference: B. Kriete, J. Lüttig, T. Kunsel, P. Malý, T. L. C. Jansen, J. Knoester, T. Brixner, and M. S. Pshenichnikov, Interplay between Structural Hierarchy and Exciton Diffusion in Artificial Light Harvesting, Nature Communications , 10 October 2019
Last modified: | 22 October 2019 09.42 a.m. |
More news
-
13 May 2024
Trapping molecules
In his laboratory, physicist Steven Hoekstra is building an experimental set-up made of two parts: one that produces barium fluoride molecules, and a second part that traps the molecules and brings them to an almost complete standstill so they can...
-
29 April 2024
Tactile sensors
Every two weeks, UG Makers puts the spotlight on a researcher who has created something tangible, ranging from homemade measuring equipment for academic research to small or larger products that can change our daily lives. That is how UG...
-
16 April 2024
UG signs Barcelona Declaration on Open Research Information
In a significant stride toward advancing responsible research assessment and open science, the University of Groningen has officially signed the Barcelona Declaration on Open Research Information.