MSc and BSc projects
There are always exciting opportunities for talented Master and Bachelor students in our team. Below, you find two example MSc projects, currently available. More MSc and BSc projects with similar focus are always possible. For more information, please contact Thomas Schlathölter.
MSC projects 2021/2022
Development of a Traveling Wave Ion Mobility Spectrometry Stage for Mass Spectrometric Studies
Mass spectrometry is a century old analytical technique applied to a wide variety of fields, from trace gas analysis – for instance, in detection of explosives in airports – to investigations of contents of planets, comets and other celestial bodies. There are many mass spectrometric sub-techniques, but all of them share a basic principle: solid, liquid or gaseous samples are positively or negatively ionized and accelerated by electric or magnetic fields. Ions with different mass-per-charge ratios (m/q) undergo different accelerations. In Time-of-flight mass spectrometry this can be seen as a molecular “race”, where lighter molecules reach the finish line before the heavier ones. At this point, a detector registers the number of ions of a specific m/q that arrived in a specific time. The result of the race is analyzed and presented as a spectrum showing intensity of the detected ion versus the m/q.
Albeit widely used and very powerful, conventional mass spectrometry has a limitation: as it is sensitive only to the m/q of the ions, it cannot distinguish species with different conformations, i.e., ions with the same m/q and different spatial positioning of its constituent atoms. This is a serious constraint, as studies of many important chemical reactions must take conformations into account, not only because some of them only occur with specific conformers, but also because environmental effects like temperature, pH and radiation levels can modify molecular three-dimensional arrangements, changing reaction paths. For instance, a slight change in an enzyme side chain can promote molecular rearrangement due to intramolecular interactions that can result in inactivation by alteration of the molecular “lock and key” profile, ultimately preventing the maintenance of life.
To address this, a family of techniques was developed under the name Ion Mobility Spectrometry (IMS). The most basic example is the Drift tube Ion Mobility Spectrometry (DT-IMS), where ions are accelerated by electric fields in a region filled with gas. The ions are still racing, but this time the process can be compared to skydiving: collisions with the gas decelerate the molecules. The most compact ones undergo less collisions and are decelerated less, being detected first, while the ones that occupy a bigger area arrive later, just as if they have a parachute open. It is, then, possible to separate conformer mixtures based on the arrival time at the end of the collision region. However, this technique also has undesirable characteristics: it requires long linear skydiving distances and high voltages to drive the molecules through this long path.
At the Quantum Interaction and Structural Dynamics (QISD) group these shortcomings are being delt with via implementation of an experimental setup that makes use of another technique, called Travelling Wave Ion Mobility Spectrometry (TW-IMS). This variation of the IMS utilizes electric potentials to create waves that push the molecules through a gas-filled region. Now, instead of skydiving, the molecules “surf” on these potential waves and the separation occurs when the ones that suffer more collisions roll over the wave crest while the ones that collide less keep up with it, effectively surfing on them. Instead of long paths, TW-IMS allows a much more compact setup, making use of printed circuit boards to generate the waves, effectively making a snake-like conveyor belt where the molecules travel. The need of high voltages is also suppressed, as successive interactions between the molecules and the waves promote separation, without the usage of one huge potential difference.
Structural characterization of clusters of amino acids
The physical, chemical and biological properties of a molecule are often closely related to its three-dimensional structure. A good example are proteins in living cells where function follows form: Only in the correct state of folding can a protein fulfill its biological task.
The most common experimental technique to separate molecular conformations in the gas-phase is ion-mobility spectrometry (IMS). The established implementation is to drag molecular ions through a gas over distances of several meters using high voltages. Over the last two years, master students in our group have implemented an advanced IMS technology that is based on traveling waves, provided by electrodes on printed circuit boards (PCBs). The figure below shows the bottom PCB (design: Jente Damm). The top PCB was removed for this photo.
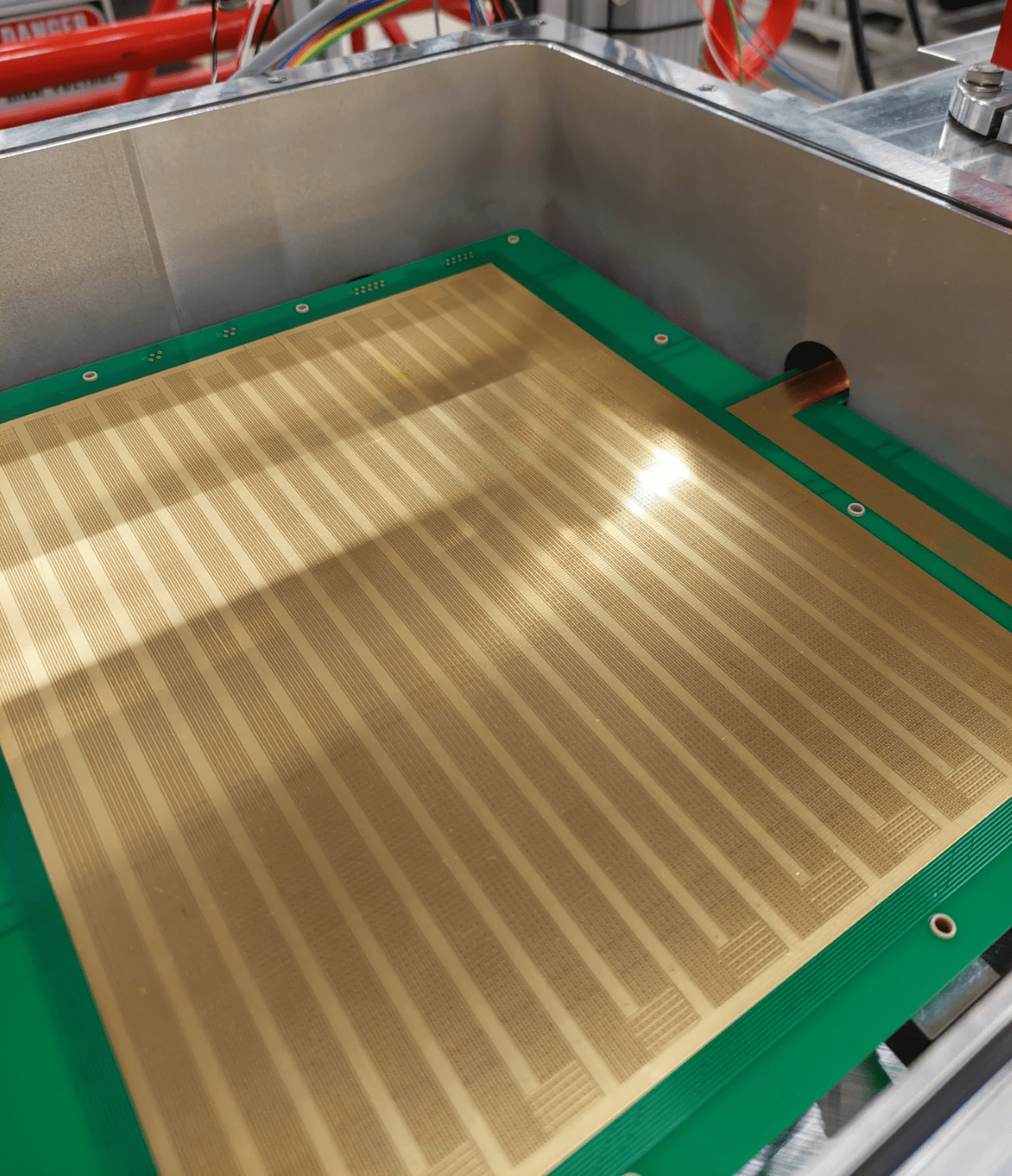
The back-and-forth marble-run provided by the PCB consists of continuous radiofrequency electrodes for ion guiding with pixelated traveling wave electrodes in between. The ions are forced to drift over a distance of about 5 meters on a 30x30 cm2 footprint.
In this project, you will perform the first scientific experiments on our new machine which will be in the context of the origin of life on earth and in the universe (http://www.olife-programme.eu/). Amino acids are commonly found in meteorites and therefore widely believed to exist in astronomical environments. It is conceivable that proteins can form in these environments from clusters of amino acids. It will be your task to study the structural diversity of clusters of simple amino acids by means of IMS.
MSC project 2021 – Multiple electron detachment Auger decay in single DNA molecules
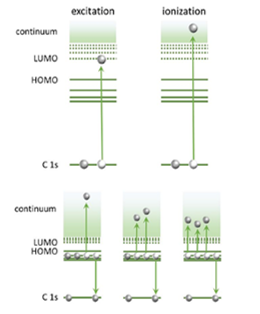
One of the most fundamental processes underlying radiotherapy is the radiation-induced production of low energy electrons in biological systems. These electrons in turn are responsible for a very large part of the biological damage, in particular to cellular DNA.
In our group we have recently shown that X-rays as well as (therapeutically relevant) MeV ions both lead to removal of several electrons in single collisions with isolated DNA molecules. This is a very surprising result, as the underlying interaction process is inner-shell excitation or ionization. The induced inner-shell vacancy then decays by a so-called Auger process in which most of the excitation energy was expected to be released in the form of kinetic energy of a single emitted Auger electron. Our results indicate that in this process at least 3 electrons are emitted, which has fundamental implications for our understanding of biological radiation damage.
In this project, you will use our gas-phase biomolecule apparatus to prepare targets of synthetic DNA anions in a radiofrequency ion trap. During an experimental campaign at the SOLEIL synchrotron in Orsay, you will directly measure the Auger electrons emitted after X-ray interactions with these trapped DNA anions.
MSc project 2021: Hydrogenation of N-substituted PAHs
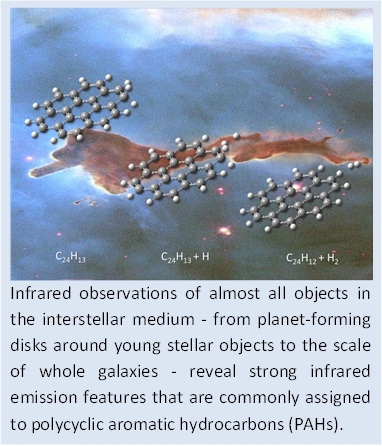
Molecular hydrogen is the most abundant molecule of our Universe. H2 formation on Polycyclic Aromatic Hydrocarbon (PAH) cations is considered a potentially important route towards molecular hydrogen formation in the interstellar medium (ISM). Hydrogen interaction with PAHs furthermore is a model system for hydrogen-graphene interactions, the latter being key for future graphene-based hydrogen storage appliactions.
We have recently found that for coronene cations (C24H12 +), sequential H attachment involves well defined adsorption sites. Along this sequence, "magic numbers" of attached H atoms are observed to be particularly stable [1]. The process competes with H2 abstraction, where an incoming H atom reacts with a previously attached H atom, to form a free H2 molecule.
Until now, attachment and abstraction reactions have only been studied for genuine polycyclic aromatic hydrocarboncations. In this project, the process will be investigated systems where single C atoms are substituted by N. These systems are relevant from an astrobiological point of view, as they could be a template for formation of prebiotic molecules in astrophysical environments.
[1] S. Cazaux, L. Boschman, N. Rougeau, G. Reitsma, R. Hoekstra, D. Teillet-Billy, S. Morisset, M. Spaans & T. Schlathölter, Nature Scientific Reports 6 (2016) 19835
MSc projects: Soft X-ray and heavy ion interactions with gas-phase DNA
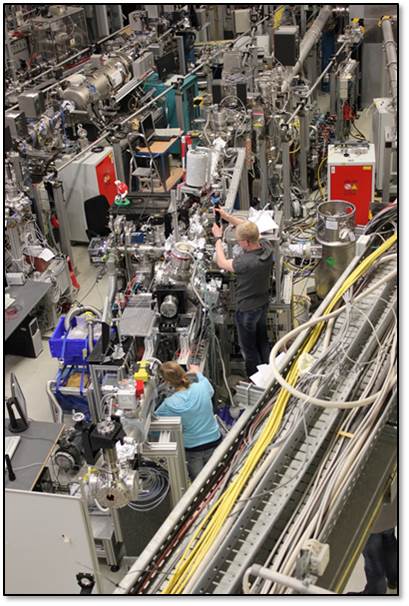
The concept of radiotherapy for tumor control is based on the idea of killing cancerous cells by means of ionizing radiation, while simultaneously sparing healthy surrounding tissues. This implies delivery of high doses to tumour volumes while minimising dose deposition into surrounding healthy tissues. To increase the contrast between dose to tumor and healthy tissue so-called radiosensitizers are enriched in the tumor. Heavy metal nanoparticles are the most recent group of radiosensitizers, for which the clinical potential is currently investigated. Currently, the underlying mechanisms on a molecular level are not fully understood for X-ray irradiation and even more ambiguous for proton or heavy ion therapy. The investigation of this issue in macroscopic systems (in vivo and in vitro) is difficult because of the complexity of the systems and because timescales range from the fs-scale of primary ionization and excitation processes over ms timescales of diffusion limited radical chemistry up to second and even year timescales of biological processes and biological endpoints.
In our group, we are investigating the most fundamental processes of radiation action on DNA on a molecular level, i.e. on sub-nanometer length-scales and on few femtosecond time-scales. In the course of this project, you will use a radiofrequency ion trap, to prepare a well defined target of gas-phase DNA ions. You will then study the interaction of soft X-rays with these targets during an experimental campaign (typically two weeks) either at the synchrotron BESSY II (Berlin) or SOLEIL (Paris).
Last modified: | 09 May 2022 12.23 p.m. |