Research
Nanophotonics and molecular electronics are rapidly evolving fields with many promising applications ahead. The challenge is to identify suitable materials and nanostructures to enable or to optimise the desired functionality. The research in our group focuses on organic functional materials and supramolecular (nano)systems that are relevant for these fields. We want to understand how energy flows through such system, how light propagates within nanostructures, how we can actively control energy flow and / or light propagation, and in general we want to learn about the optical and electronic properties of these materials. Ultimately, our work should help to design new, more efficient architectures, e.g. for nanophotonic applications and light-energy conversion based on organic molecules.
On the material side we study conjugated polymers / oligomers, low-molecular weight organic molecules and supramolecular structures thereof. As methods we employ a broad range of complementary optical spectroscopy and microscopy techniques both on the ensemble and single molecule (single object) level. If required, we combine experiment with numerical modelling.
Conjugated Polymers/Oligomers
Conjugated polymers – often referred to as organic semiconductors – represent an important class of functional materials with applications in e.g. organic solar cells, sensors, and field-effect transistors. Our work on this material class focusses on two areas:
-
We aim at establishing structure-function relationships at a truly molecular level: We want to understand how the photophysics is determined by the chemical structure of the backbone and the side chains as well as by the conformation of the conjugated backbone.
- We study energy transport dynamics and light propagation properties within organic supramolecular nanostructures based on conjugated oligomers and polymers: We want to understand how the packing and the order (structural, electronic) within such structures influences energy transport and light propagation.
Our work on these functional materials and structures will help to obtain a clearer picture of the various processes occurring in real device applications, and may help to identify strategies for optimisation of device performance.
Structure-Function Relationships in Single Oligo- and Polythiophene Chains
We investigated the prototypical conjugated polymer poly(3-hexyl-thiophene), P3HT, using low-temperature single-molecule photoluminescence spectroscopy,. We found that individual chains are structurally surprisingly ordered, with only small variations of dihedral angles between thiophene units around the mean angle (35 °). A further important observation was the strong tendency of P3HT to form chain-chain contacts by van-der-Waals interactions between the aromatic backbones (Fig. 1). These give rise to the formation of ‘loose aggregates’ even at very low (nanomolar!) concentrations in the best solvents known for this polymer. The photoluminescence of these ‘loose aggregates’ entirely dominates in any P3HT-solution as well as in disordered P3HT-films.
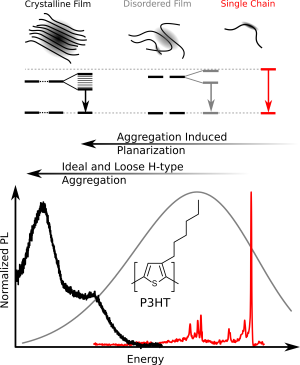
Moreover, we studied how the typically flexible side chains appended to the backbone influence the photophysics of conjugated polymers. So far the side chains were thought to exclusively provide solubility. However, we were able to demonstrate that tailored side chains can control the backbone conformation. For instance, we replaced the short side chains of P3HT (hexyl) by very bulky ones (dioctylphenyl) to obtain the polythiophene-derivative PDOPT. We found that the bulky side chains form non-covalent interactions with the thiophene-backbone: The side chains “embrace” the backbone and form structures resembling barber’s pole stripes (Fig. 2, top). This “embracing” planarises the backbone, and gives rise to a large 70 nm red-shift of the photoluminescence (Fig. 2, bottom), i.e., more than 10 % of the optical gap! In other words, simply by changing the side chains, the photoluminescence can be shifted from the green part of the visible spectral range (P3HT) to the reddish part (PDOPT) without elaborate backbone chemistry. This side-chain engineering may provide a new route for the colour-tuning of organic displays.
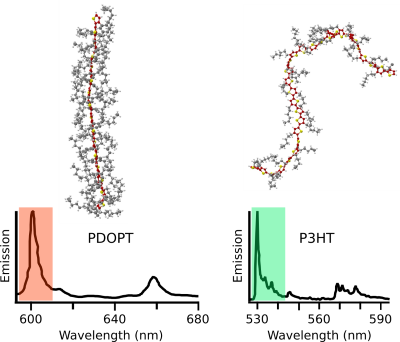
Top right: P3HT with short hexyl side chains exhibits its characteristic bent (Courtesy Lena Simine, Rice University).
Bottom: Single-molecule photoluminescence spectra of single PDOPT (left) and P3HT (right) chains. Adapted from Raithel et al. PNAS 2018.
References:
Raithel, D.; Simine, L.; Pickel, S.; Schötz, K.; Panzer, F.; Baderschneider, S.; Schiefer, D.; Lohwasser, R.; Köhler, J.; Thelakkat, M.; Sommer, M.; Köhler, A.; Rossky, P. J.; Hildner, R. Direct Observation of Backbone Planarization via Side-Chain Alignment in Single Bulky-Substituted Polythiophenes. Proc. Natl. Acad. Sci. U.S.A. 2018, 115, 2699–2704.
Raithel, D.; Baderschneider, S.; de Queiroz, T. B.; Lohwasser, R.; Köhler, J.; Thelakkat, M.; Kümmel, S.; Hildner, R. Emitting Species of Poly(3-Hexylthiophene): From Single, Isolated Chains to Bulk. Macromolecules 2016, 49, 9553-9560.
Cooperation Partners:
Mukundan Thelakkat (Bayreuth)
Michael Sommer (Chemnitz)
Anna Köhler (Bayreuth)
Stephan Kümmel (Bayreuth)
Thiago de Queiroz (Santo André, Brasil)
Lena Simine (Rice University; McGill University, Montreal)
Peter Rossky (Rice University)
Light Propagation in Single Crystals Based on Oligothiophenes
Conjugated polymers and oligomers are also useful building blocks for the self-assembly into ( m m- to mm-sized) single crystals (Fig. 3, left). Employing Fourier-space microscopy and spectroscopy, we investigate the light propagation (active waveguiding) within such highly anisotropic systems. For instance, the self-interference of light waveguided over different distances can be exploited to visualise the dispersive behaviour of the anisotropic refractive index (Fig. 3, right). Moreover, we can show how the refraction of (waveguided) light at nanoscale edges deviates from classical ray optics.
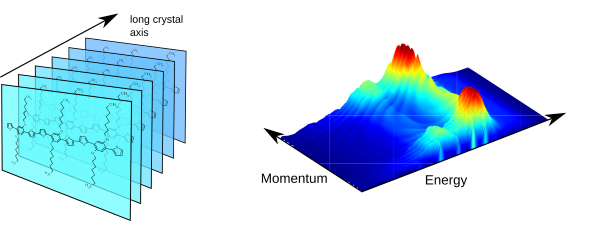
References:
Schörner, C.; Motamen, S.; Simon, L.; Reiter, G.; Hildner, R. Self-Interference of Exciton Emission in Organic Single Crystals Visualized by Energy-Momentum Spectroscopy. ACS Omega 2018, 3, 6728–6736.
Motamen, S.; Schörner, C.; Raithel, D.; Malval, J.-P.; Jarrosson, T.; Serein-Spirau, F.; Simon, L.; Hildner, R.; Reiter, G. Low Loss Optical Waveguiding in Large Single Crystals of a Thiophene-Based Oligomer. Phys. Chem. Chem. Phys. 2017, 19, 15980–15987
Cooperation Partners:
Hans-Werner Schmidt (Bayreuth)
Günter Reiter (Freiburg)
Laurent Simon (Mulhouse)
Supramolecular Nanofibres Based on Small Organic Molecules
A second major research topic in our group is the investigation of the transport of electronic excitations along well-defined supramolecular nanostructures. This transport is a fundamental process to understand the initial light-driven steps in artificial and natural structures for light-energy conversion, e.g. in photosynthetic organisms. We aim at establishing design principles to create supramolecular structures that are optimised for highly efficient, long-range energy transport.
As building blocks we use carbonyl-bridged triarylamine-derivatives with specific supramolecular motifs ( π-π interactions, hydrogen-bonding groups). We achieved robust self-assembly of these building blocks into micrometre-long, well-defined isolated nanofibres (Fig. 4). The dense packing facilitates strong electronic interactions between the building blocks, that allowed us to demonstrate record-breaking partially coherent energy transport over > 4 µm along individual nanofibres at room temperature.
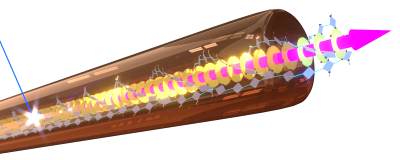
References:
Brixner, T.; Hildner, R.; Köhler, J.; Lambert, C.; Würthner, F. Exciton Transport in Molecular Aggregates - From Natural Antennas to Synthetic Chromophore Systems. Adv. Energy Mater. 2017, 7, 1700236.
Haedler, A. T.; Kreger, K.; Issac, A.; Wittmann, B.; Kivala, M.; Hammer, N.; Köhler, J.; Schmidt, H.-W.; Hildner, R. Long-Range Energy Transport in Single Supramolecular Nanofibres at Room Temperature. Nature 2015, 523, 196–199.
Cooperation Partners:
Hans-Werner Schmidt (Bayreuth)
Milan Kivala (Heidelberg)
Eva Herzig (Bayreuth)
Last modified: | 12 November 2018 11.55 a.m. |