Research
Why?
In order to meet the global energy need in a sustainable way alternative sources of energy need to be developed. This is largely a matter of finding better materials, processes and device structures. Our field, device physics, contributes to this quest by identifying which materials properties need to be improved in order to improve performance (efficiency, stability, cost). A close collaboration between chemistry and physics is required to make progress.
How?
We use a combination of experimental and simulation techniques to identify how the performance of devices is related to the properties of the materials and basic physical processes. Our work focuses on functional materials that can be easily processed from solution. This means that using the materials to make a functional device requires little energy and is cheap.
What?
We work on and organic thermoelectrics, perovskite solar cells, and organic solar cells.
Organic thermoelectrics
In the quest for renewable energy, the possibility to recover waste heat must not be overlooked. About one third of the energy used by industry is turned into waste heat, and disposed of in the form of hot exhaust gases, cooling water, etcetera. Thermoelectric generators can convert such waste heat to electricity without any moving parts by creating a voltage when there is a different temperature on each side. Efficient thermoelectric generators require materials with high conductivity and Seebeck coefficient while having low thermal conductivity. Organic semiconductors have very low thermal conductivity making them suitable for thermoelectrics. However, in order to compete with traditional inorganic materials more research is needed.
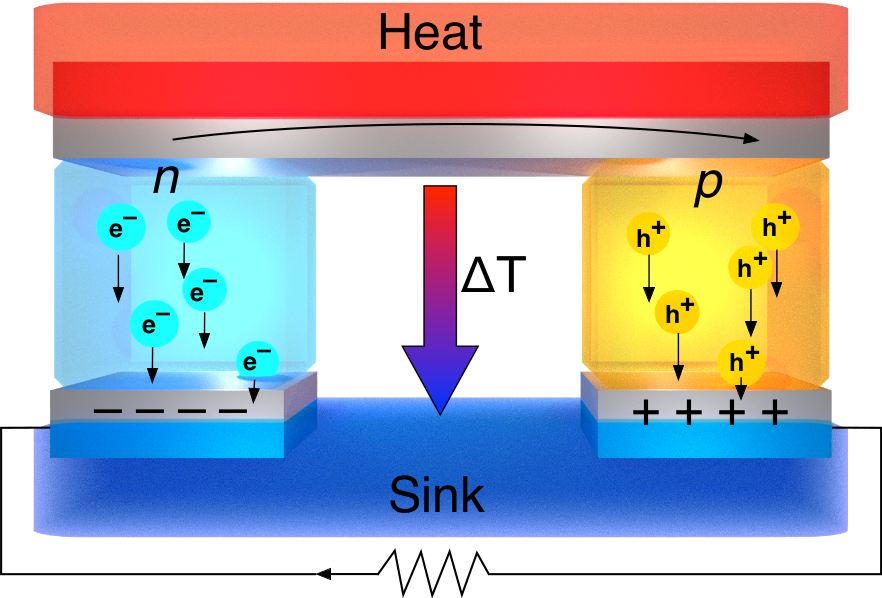
In our recent work, we have made significant progress towards a much-wanted goal: combining the unique benefits of organic semiconductors with the ease of doping of inorganics like silicon. By tailoring the side-chains on organic semiconductors, we have reached a much improved host-dopant miscibility which has lead to superior doping efficiencies (increased by 1 order of magnitude) and conductivity (increased by 2 orders of magnitude) [1-4]. We have shown that this strategy works for both small molecules [2-4] and conjugated polymers [1]. This approach not only improves the doping efficiency by better miscibility, but the polar side chains also screen the Coulomb interaction between dopants and free charge carriers. This makes the doping process itself—regardless the miscibility—more efficient [5]. Improved interactions between the host and the dopant also lead to better microstructure, making the doped films more stable against heat [2] and air [4].
Perovskite solar cells
Perovskite solar cells have attracted significant attention in the last few years due to the unparalleled improvements in efficiency. However, the understanding of the device physics is still limited. We use a combination of experimental and theoretical techniques to shed light on their workings.
![Fig. 2 We recently showed [6] that there is a direct correlation between the density of traps, mobile ions, and the hysteresis in perovskite solar cells.](/staff/l.j.a.koster/toc_tejas.png)
In a recently published paper [6], we showed that the main losses in the perovskite solar cells studied occur at the interfaces between the perovskite and the charge transport layers, ruling out the scenario of strong bulk trap-assisted recombination in the perovskite. We observe direct correlation between the density of traps, the density of mobile ionic defects, and the degree of hysteresis in perovskite solar cells.
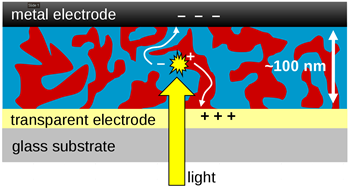
Organic solar cells
The organic materials used in organic solar cells are conjugated. This makes them semiconducting. Organic solar cells potentially have many advantages over their inorganic counterparts―such as cheaper manufacturing, and being flexible and lightweight―but their efficiency has still been much less than inorganic solar cells. Recent developments in the field make organic solar cell still promising with record efficiencies of 15.7% for single junction and 17.3% for multijunction cells.
However, for any technology to be commercially viable, it must meet the golden triangle rule (Efficiency, Reliability/Stability, and Cost): Increase efficiency and stability but keeping the cost low. While efficiency and cost have been boosted over the years, stability is somehow stagnated or lagging far behind. Therefore, this area has been the focus of our recent efforts.[7–10] In direct collaboration with chemists, we have demonstrated how strongly related the degradation of organic solar cells is to the chemical structure of the organic materials employed in the active layer blends. We have identified through chemistry and device physics the process of the degradation mechanism that occurs in polymer solar cells whether fullerene-based or non-fullerene-based cells. In so doing, we able to pinpoint the origin of the observed instability in the cells which is mostly linked to the chemical structure of the molecules: side chains, backbones, and the addition or removal of electronegative atoms (such as O, F, Cl etc.) or electron-rich group like methyl (-CH3) .
References
[1] J. Liu, L. Qiu, R. Alessandri, X. Qiu, G. Portale, J. Dong, W. Talsma, G. Ye, A.A. Sengrian, P.C.T. Souza, M.A. Loi, R.C. Chiechi, S.J. Marrink, J.C. Hummelen, and L.J.A. Koster, Adv. Mater. 1704630 (2018).
[2] J. Liu, L. Qiu, G. Portale, M. Koopmans, G. ten Brink, J.C. Hummelen, and L.J.A. Koster, Adv. Mater. 1701641 (2017).
[3] L. Qiu, J. Liu, R. Alessandri, X. Qiu, M. Koopmans, R.W.A. Havenith, S.J. Marrink, R.C. Chiechi, L.J.A. Koster, and J.C. Hummelen, J. Mater. Chem. A 5, 21234 (2017).
[4] J. Liu, L. Qiu, G. Portale, S. Torabi, M.C.A. Stuart, X. Qiu, M. Koopmans, R.C. Chiechi, J.C. Hummelen, and L.J.A. Koster, Nano Energy 52, 183 (2018).
[5] J. Liu, S. Maity, N. Roosloot , X. Qiu, L. Qiu, R. C. Chiechi , J. C. Hummelen , E. von Hauff, and L.J.A. Koster, Adv. Electronic Mater (accepted).
[6] T.S. Sherkar, C. Momblona, L. Gil-Escrig, J. Ávila, M. Sessolo, H. Bolink, and L.J.A. Koster, ACS Energy Lett. 2, 1214 (2017).
[7] N. Y. Doumon, G. Wang, R. C. Chiechi, L. J. A. Koster, J. Mater. Chem. C 5, 6611 (2017).
[8] N. Y. Doumon, M. V Dryzhov, F. V Houard, V. M. Le Corre, A. R. Chatri, P. Christodoulis, L.J.A. Koster, ACS Appl. Mater. Interfaces, DOI 10.1021/acsami.8b20493 (2019).
[9] N. Y. Doumon, L. J. A. Koster, Sol. RRL 1800301 (2019).
[10] N. Y. Doumon, G. Wang, X. Qiu, A. J. Minnaard, R.C. Chiechi, L.J.A. Koster, Scientific Reports (accepted).
![g. 3. The fill-factor (FF) of many different organic solar cells plotted versus the ratio of extraction to recombination rate (θ) [3].](/research/zernike/photophysics-and-opto-electronics/koster-group/fig2_ff_theta_350.png)
Last modified: | 01 March 2024 11.25 a.m. |