Waarom bliksem vaak meermaals inslaat
In tegenstelling tot wat vaak wordt gedacht, slaat bliksem vaak meermaals in, maar de reden waarom een bliksemkanaal ‘hergebruikt’ wordt, is tot nu toe onopgehelderd. Recentelijk heeft een internationaal onderzoeksteam onder leiding van de Rijksuniversiteit Groningen (RUG) gebruik gemaakt van de LOFAR radiotelescoop om de ontwikkeling van bliksemflitsen in ongekend detail te bestuderen. Hun werk laat zien dat de negatieve lading van een onweerswolk niet in zijn geheel in één enkele flits ontladen wordt, maar dat een deel ervan kort wordt opgeslagen langs het plasmakanaal nabij breekpunten. Dit gebeurt in structuren die nooit eerder beschreven zijn, door de onderzoekers ‘naalden’ genoemd. Door deze naalden kan een negatieve lading resulteren in een herhaaldelijke ontlading op de aardbodem. De resultaten zijn op 18 april in het wetenschappelijke tijdschrift Nature gepubliceerd.
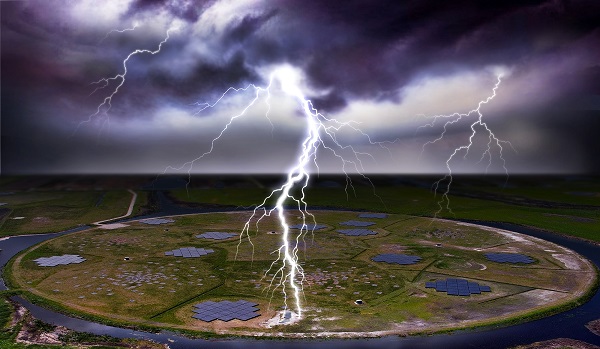
“Deze bevinding staat haaks op het huidige beeld, waarin de lading langs plasmakanalen vloeit en direct of van één deel van de wolk naar een ander deel gaat, of naar de aardbodem”, legt Olaf Scholten uit, hoogleraar Natuurkunde aan het KVI-CART van de Rijksuniversiteit Groningen. De reden waarom de naalden nooit eerder zijn waargenomen, ligt in de ‘topcapaciteit’ van LOFAR, voegt zijn collega dr. Brian Hare toe, de eerste auteur van het artikel: “Deze naalden kunnen een lengte hebben van 100 meter en een diameter van minder dan vijf meter, en zijn te klein en te kortstondig om door andere bliksemdetectiesystemen geregistreerd te worden.”
De Low Frequency Array (LOFAR) is een Nederlandse radiotelescoop die bestaat uit duizenden redelijk eenvoudige antennes die verspreid zijn over Noord-Europa. De antennes zijn met glasvezelkabels verbonden aan een centrale computer, wat betekent dat ze als één geheel kunnen functioneren. LOFAR is voornamelijk ontwikkeld voor het doen van radioastronomische waarnemingen, maar het frequentiebereik van de antennes maakt het systeem ook geschikt voor bliksemonderzoek, omdat bliksemontladingen sterke pulsen uitzenden in de VHF-radiofrequentieband (zeer hoge frequentie).
Binnen in de wolk
Voor de bliksemwaarnemingen in het nieuwe onderzoek hebben de wetenschappers alleen van de LOFAR-stations op Nederlands grondgebied gebruik gemaakt, die samen een oppervlak van 3.200 vierkante kilometer beslaan. Er is een analyse gemaakt van de ruwe tijdsporen (die tot op een nanoseconde nauwkeurig zijn), gemeten in de 30-80 MHz-frequentieband. Brian Hare: “Met deze gegevens kunnen we de verplaatsing van de bliksem op een dusdanige schaal bekijken dat we, voor het eerst, in staat zijn om de primaire processen te onderscheiden. Bovendien geeft het gebruik van radiogolven ons de mogelijkheid om binnen in de wolk te kijken, waar het merendeel van de bliksem zich bevindt.”
Bliksem ontstaat wanneer sterke opwaartse luchtstromen een soort statische elektriciteit opwekken in grote cumulonimbuswolken. Delen van de wolk raken positief geladen en andere delen juist negatief. Wanneer deze ladingsscheiding sterk genoeg is geworden, vindt er een heftige ontlading plaats, die we als bliksem kennen. Een dergelijke ontlading begint ermee dat er een plasma ontstaat, een klein gebied van geïoniseerde lucht dat zo heet is dat het elektrisch geleidend wordt. Dit kleine gebied groeit dan uit tot een gevorkt plasmakanaal dat meerdere kilometers lang kan worden. De positief geladen uiteinden van het plasmakanaal verzamelen uit de wolk negatieve ladingen, die zich door het kanaal verplaatsen naar het negatief geladen uiteinde, waar de lading gedeponeerd wordt. Het was al bekend dat er aan de kant van de groeiende uiteinden van de negatieve kanalen een grote hoeveelheid VHF-emissie geproduceerd wordt, terwijl de positieve kanalen alleen emissies vertonen langs het kanaal, en niet aan het uiteinde ervan.
Een nieuw algoritme
De wetenschappers hebben een nieuw algoritme voor de LOFAR-gegevens ontwikkeld, waardoor ze van twee bliksemflitsen de VHF-radio-emissies konden visualiseren. Dankzij de verspreiding van de antennes over een groot gebied en het zeer nauwkeurige tijdstempel op alle vergaarde gegevens, konden ze de bronnen van de emissie met een niet eerder vertoonde precisie lokaliseren. “Dicht bij het kerngebied van LOFAR, waar de antennedichtheid het grootst is, was de ruimtelijke nauwkeurigheid ongeveer een meter”, zegt professor dr. Scholten. Bovendien was het met de verzamelde gegevens mogelijk om tien keer zoveel VHF-bronnen te lokaliseren dan met andere driedimensionale beeldvormingssystemen, met een tijdsresolutie in de orde van nanoseconden. Dit resulteerde in een hoge resolutie 3D-beeld van de bliksemontlading.
Breekpunt
De resultaten laten duidelijk zien dat er in het ontladingskanaal een breekpunt optreedt, op een locatie waar naalden gevormd worden. Deze lijken negatieve ladingen uit het hoofdkanaal op te nemen, die vervolgens terug de wolk in gaan. De vermindering van de lading in het kanaal is wat de breuk veroorzaakt. Maar zodra de lading in de wolk weer hoog genoeg wordt, wordt de stroom door het kanaal hersteld, wat zich uit in een tweede ontlading. Door dit mechanisme slaat de bliksem herhaaldelijk in hetzelfde gebied in.
Scholten: “De VHF-emissies langs het positieve kanaal zijn het gevolg van vrij regelmatig herhaalde ontladingen in eerder gevormde zijkanalen, de naalden. Het lijkt zo te zijn dat deze naalden de lading met een gepulseerd ritme wegvoeren.” Dit is een volledig nieuw verschijnsel, voegt professor Joe Dwyer van de University of New Hampshire (VS) toe, de derde auteur van het artikel: “Onze nieuwe waarnemingsmethoden maken in de bliksemflits talloze naalden zichtbaar die niet eerder te zien waren.” En Brian Hare concludeert: “Deze waarnemingen laten zien hoe een deel van de wolk opnieuw geladen wordt, en ze maken begrijpelijk waarom de bliksemontlading naar de grond een paar maal herhaald kan worden.”
Informatie
Referentie: Brian Hare, Olaf Scholten, Joe Dwyer, et al. Needle-like structures discovered on positively charged lightning branches. Nature, 18 April 2019.
DOI: 10.1038/s41586-019-1086-6
Van de reële waarnemingen die voor dit paper gebruikt zijn, zijn twee video's gemaakt:
- Video 1: Bliksem – compleet
De video toont in slow-motion de ontwikkeling van de bliksemschicht. In werkelijkheid duurt deze minder dan 0,2 seconden en tegen het einde van de ontlading strekt deze zich in alle richtingen over ongeveer vijf kilometer uit. De felgele flitsen geven de nieuw gedetecteerde radiopulsen aan, die al snel vervagen tot kleine witte puntjes, om een idee van de structuur te geven. De positief geladen bliksemkanalen zijn aan de bovenkant van de bliksemflits te zien. Deze groeien naar boven en lijken door de nieuw ontdekte naalden te fonkelen. Het fonkelen vindt vooral plaats tussen 0:14 en 0:21 seconden. De negatief geladen kanalen groeien naar beneden en er is te zien dat ze steeds blijven groeien (deze fonkelen niet). Na 0:14 seconden bereikt een van de negatieve kanalen zelfs de grond. Tegen het einde van de video vindt er nog steeds wat activiteit plaats langs de loop van het positieve kanaal. - Video 2: Bliksem – naald
De video laat een ingezoomd beeld zien van het positief geladen kanaal. In werkelijkheid duurt het maar 0,1 seconde en beslaat het afgebeelde gebied een afstand van ongeveer 400 meter. Aanvankelijk is er weinig activiteit te zien langs het kanaal: er is nauwelijks iets zichtbaar. Pas na 0:10 seconden zie je dat er zich naalden ontwikkelen. Een bijzonder grote naald is in het rood afgebeeld. Langs het bliksemkanaal zijn er meerdere naalden te zien en je kunt elke naald, inclusief de rode, meerdere keren zien ‘oplichten’. Na 0:20 seconden houdt de activiteit in dit deel van het kanaal op, terwijl er op verder weg gelegen punten langs het kanaal nog wat activiteit plaatsvindt.
Video-credits: Stijn Buitink (VUB, Brussel) en Brian Hare (Rijksuniversiteit Groningen)
Laatst gewijzigd: | 11 mei 2020 13:26 |
Meer nieuws
-
06 juni 2025
India-Nederland Hydrogen Valley Fellowship-programma aangekondigd
Ter gelegenheid van Wereldmilieudag op 5 juni 2025 maakten het Indiase ministerie van Wetenschap en Technologie en de RUG gisteren de start bekend van een partnerschap voor het Hydrogen Valley Fellowship-programma. Dit programma biedt getalenteerde...
-
05 juni 2025
Planten die hun eigen hachje redden
Kira Tiedge onderzoekt de chemische stoffen waarmee planten met hun omgeving communiceren, om zo robuuste gewassen te selecteren die beter bestand zijn tegen bijvoorbeeld ziekte en droogte.
-
04 juni 2025
Dode Zeerollen ouder dan gedacht
Veel Dode Zeerollen zijn ouder dan eerder werd gedacht. En niet alleen dat: ook blijkt dat twee fragmenten van de bijbelse rollen uit de tijd stammen van hun vermoedelijke auteurs. Dit constateert een internationaal team van onderzoekers onder...