Research
Current research directions:
I) Antiferromagnetic Spintronics with complex oxides
Multiferroics have found renewed interest particularly for topological magnetism and for logic-in-memory applications such as in MESO demonstrated by Intel and in FESO devices. The coexistence and coupling between electricity and magnetism makes multiferroic materials highly desirable both for fundamental exploration as well as for such technological applications.
-
We have been working on SrMnO3 (SMO) which is a rare example of a multiferroic, theoretically predicted to exhibit a very strong magnetoelectric coupling due to strain. Strain control can lead to a suite of antiferromagnetic states in SMO. We use electrical detection schemes for the investigation of such coexisting magnetic order. The challenge is however to grow these materials with minimal oxygen vacancies. This, we have optimized using a particular oxygenation protocol in a Pulsed Laser deposition set up. We use the technique of Spin Hall Magnetoresistance (SMR), which in recent years has become an established method to probe surface magnetic order, particularly attractive for antiferromagnets. We have tapped the potential of SMR beyond that used for conventional ferromagnets and antiferromagnets and have made fundamental contributions in understanding the competing ground states in SMO. We use the potential of SMR in reading out surface magnetic moments, find coexistence of different antiferromagnetic domains and demonstrate how we read the surface magnetic order and its spatial variation by careful device design. This is done by a detailed analysis of the amplitude and phase of the SMR -aspects which were not exploited earlier. We collaborated with researchers from Uppsala University for DFT studies on magnetic exchange and magnetic anisotropy in thin films of SMO. We are currently looking into magnon spin transport in these materials. Besides these, we are currently working on probing the ferroelectric order in these challenging materials using a suite of microscopic tools and STEM revealing important correspondence between the growth, microstructure and coupling of the ferroic orders in SMO thin films.
-
BiFeO3 is the most investigated multiferroic system today. In a collaboration with colleagues at FLEET Centre [UNSW, Australia, University of Canterbury, New Zealand and University of Arkansas, USA] we have demonstrated how crystal structure and strain engineering are crucial to understand the magnetic response in thin films of BFO on different substrates. This work also represents a major advance in antiferromagnetic spintronics and multiferroics. More details can be found here https://arxiv.org/abs/2308.12500
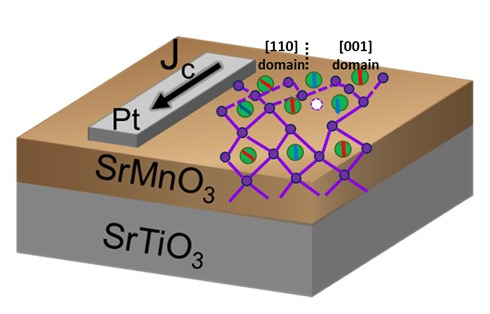
II) New pathways with Skyrmion bubbles exploiting magnetocrystalline anisotropy in SrRuO3
Magnetic bubbles are cylindrically shaped islands with reversed magnetization that can be set into motion by electric current pulses. They have been discovered in 1970’s and were immediately employed to store digital information in non-volatile memory that can withstand harsh environments. The interest in magnetic bubbles was recently spurred by the discovery that in chiral magnets (magnets different from their mirror images) their size can be reduced down to a few nanometers, which makes them excellent candidates for novel high-density magnetic memory devices. The magnetic dipoles in the nanosized bubbles, aka skyrmions, form a knot that cannot be easily unwound. This non-trivial topology gives rise to a skew-scattering of electrons off skyrmions – the so-called Topological Hall Effect. We have detected signatures of the skew scattering in the ferromagnetic conductor, SrRuO3, associated with topological magnetic bubbles, which showed a surprising adaptability to different substrate surfaces and unique resilience to variations of temperature, strength and direction of the applied magnetic field. This work was done along with the group of Maxim Mostovoy at ZIAM and researchers at the Institut für Festkörperphysik, TU Wien, Austria. Hitherto ignored higher-order magnetocrystalline anisotropy terms and the long-ranged magnetodipolar interactions were invoked to explain the much greater stability of such topological textures, allowing us to construct detailed phase diagrams. Theoretical studies traced the origin of this unexpected stability to the unusual shape of the bubbles and complex magnetic behavior of SrRuO3. These new insights opens significant opportunities to tailor device interfaces for new magnetic memory technologies and novel hardware components for alternative computing strategies.
We also extended this work using an alternative strategy related to differences in the oxygen vacancies at two different heterointerfaces of SrMnO3/SrRuO3 (SMO/SRO) by tailoring the growth conditions in SMO. We show how the control of the octahedral tilt and of magnetocrystalline anisotropy has a profound influence in the observation of Topological Hall Effect and can lead to the vanishing of THE, for devices that are less oxygen deficient.
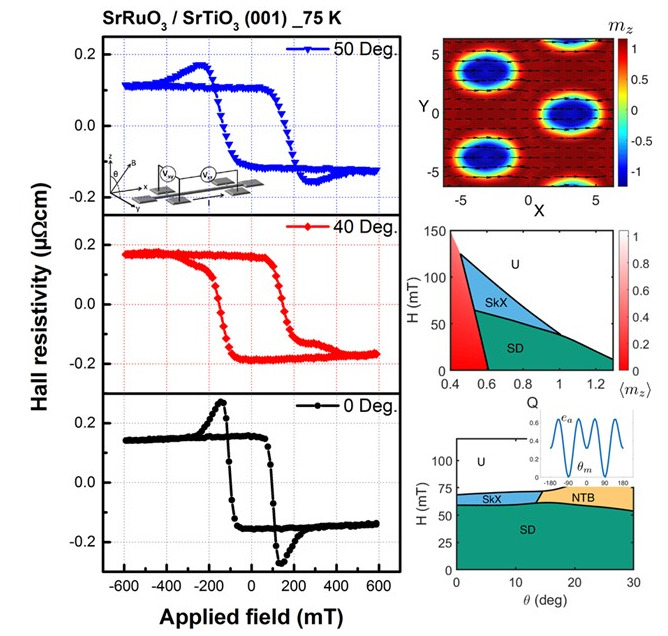
III) New memristive devices for neuromorphic computing applications: non- volatile and volatile memristors on complex oxides.
The massive increase in the amount of data generated has led to shortcomings in the ability of the current technologies to deal with the data in an energy-efficient way. Consequently, computational hardware and architectures that can process data more efficiently are highly sought after with the brain serving as a source of inspiration. This cannot be realized using von Neumann systems and hence a new avenue of neuromorphic computing calls for research into emerging devices, such as memristors. We work on memristive devices both non volatile and volatile memristors in our group using complex oxides and integrate these devices directly on a semiconducting platform.
-
We demonstrate an alternative type of memristors; interface memristors on Nb-doped SrTiO3 doped semiconductor, with non volatile memory, readily scalable, showing analog, multilevel switching without a forming step, a remarkable high memory window, low device-to-device variability and high endurance. This is enabled by device designs that leverage edge effect-related electric fields across such interfaces, allowing for the locally enhanced fields to tune the interfacial energy landscape. This was also corroborated from Scanning transmission electron microscopy (STEM) studies and device modelling done along with Majid Ahmadi in the group of Bart Kooi at ZIAM. These non volatile memristors can be programmed to store information and thus operate at different time scales by designing the external stimuli. We fabricate our devices using Pulsed laser deposition and other evaporation tools and lithography techniques existing at Nanolab Groningen.
The rapid impact of the work resulted in contributions in different areas such as in energy efficient memristive-TCAM, device models, security applications along with different CogniGron groups and we are currently working on different types of memristive devices for this.
-
Neuromorphic computing utilizes energy-efficient non-von Neumann approach, but at the hardware level it lacks rich non-linear phenomenon that occurs in biological systems. Such systems would require low energy and capable of fast neuronal spiking. We are actively working on volatile memristors using complex oxides for this. Usually volatile memristive switching is triggered either by temperature or electric field, driving the switching layer from an insulating to a metallic state, often requiring a high voltage forming step or high temperature. We have exploited the rich physics of strongly correlated systems and use new device designs relying on the morphology of the substrate that demonstrate anisotropic and spatiotemporal control over the resistive switching dynamics across different pathways. We chose LaAlO3 as the substrate and using Pulsed Laser Deposition grow strained thin films of LSMO that stabilizes competing magnetic states in the network. The strong coupling between electronic and magnetic ground states, intrinsic to these strongly correlated materials, is exploited to study resistance changes induced by an electric current close to the competing phase transition temperature along different pathways. We are exploring the distinct negative differential resistance states in our devices both from a fundamental view point as well as by designing oscillatory circuits to probe the switching dynamics from low-bias kHz to high-bias MHz. This enhances further prospects in different domains such as in reservoir computing.
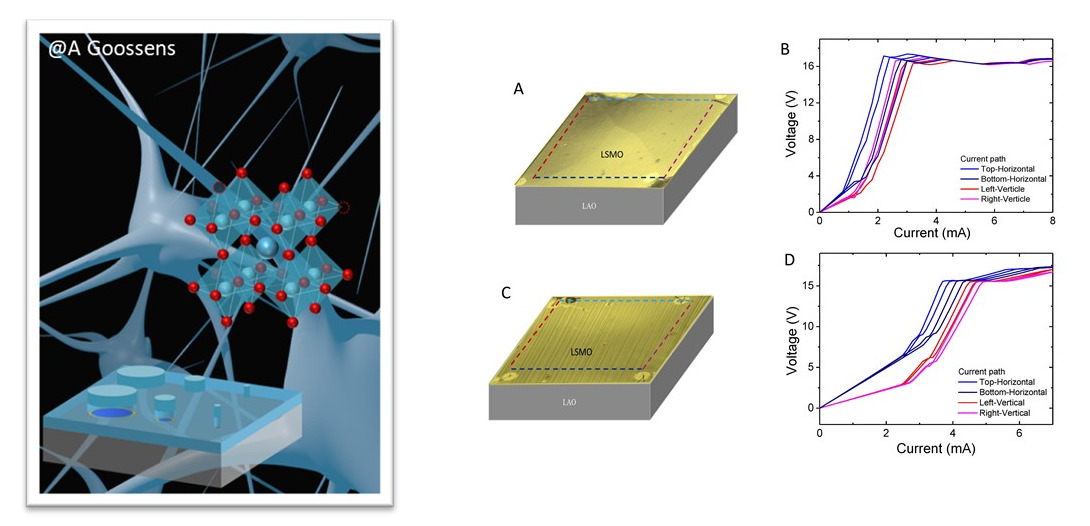
(Right) Volatile memristors using the metal-insulator transition in LSMO doi: 10.3389/fnano.2023.1121492
III) New routes in probabilistic computing
A computational primitive that is gaining rapid attention is the probabilistic bit (p-bit). The p-bit is a classical electronic entity that alternates rapidly between its allowed energy states. Unlike its deterministic counterpart - the CMOS transistor -, the output of a p-bit has stochasticity as its figure of merit and low retention times for the information stored. In such stochastic devices, the energy barrier encountered when switching between the allowed states of the system is comparable in magnitude to the thermal fluctuation. This normally undesirable feature can be exploited to not only provide analog, integrated random-number-generators on existing CMOS technologies but also serve as the building block for probabilistic circuitry. We are currently working on designing spintronics based devices for use as probabilistic bits in probabilistic hardware for solving optimization problems efficiently. For this we use complex oxides, particularly material system that exhibit rich energy landscape modulation due to competing magnetic interactions to engineer the output of the devices that can fluctuate between the 1 and 0 states. This work is done in close collaboration with computer architecture experts within CogniGron.
Few earlier works from our group:
(1) Electric field manipulation of spin transport at oxide semiconducting interfaces
We have demonstrated electric field tunability of spin lifetime in n-doped SrTiO3 at room temperature, by tailoring the potential landscape at the interface, and without the necessity of an additional gate electrode. We have also shown a new phenomenon of tunneling anisotropic magnetoresistance (TAMR) to coexist with large electroresistance effects in a ‘spin memristor’. Further, we demonstrated the coexistence of spin accumulation and TAMR at suitably engineered interfaces between doped SrTiO3 and 3d transition ferromagnets that can be tuned by temperature and applied bias. These effects originate due to the tunability of the Rashba spin orbit coupling at the interface, and their manipulation provide unique prospects for reconfigurable Spintronics architecture .
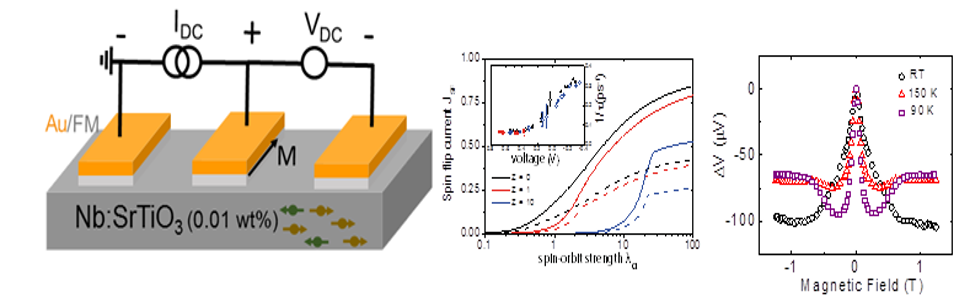
(2) Novel magnetic textures across complex oxide heterointerfaces
We explore complex magnetic textures such as bubbles and skyrmions at oxide heterointerfaces. We stabilize such textures either on i) SrRuO3/SrTiO3 or on ii) LSMO/LaAlO3 by substrate induced strain, thickness and magnetic anisotropy. We further tune the interfacial strain such that the films undergo a magnetic phase transition between antiferromagnetic insulating to a ferromagnetic metallic state and study this interplay by varying magnetic field and temperature. Using electrical transport schemes of anomalous and topological (magnon) Hall effect and a new microscopy technique of spin-filtered scanning tunneling microscopy we are currently studying different magnetic textures in such heterointerfaces, aided by micromagnetic simulations
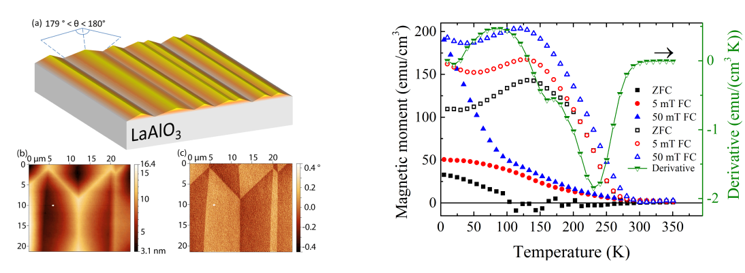
(3) Graphene spintronics on oxide interfaces
Graphene is a promising material in spintronics with predicted intrinsic spin relaxation time up to 1 µs and extremely high mobilities. In this project we used graphene as a unique platform to fingerprint the phase transition induced structural domains in STO using both charge and spin transport in graphene. We have shown how the electronically rich platform of SrTiO3 and its complex interdependence between dielectric permittivity and temperature influences spin transport in graphene and across the ferroelastic transition in SrTiO3. We are currently investigating the influence of electric field to spin transport in graphene and proximity induced exchange interactions in graphene on a multiferroic oxide substrate
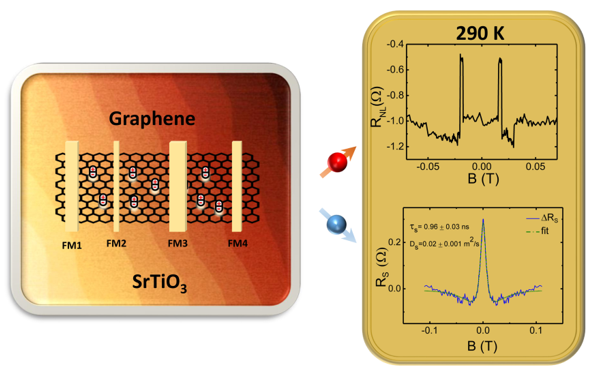
(4) Interface driven memristive devices for bio inspired computing
Most computers make use of the von Neumann architecture and, while for many tasks this computing approach works well, it cannot compete with the speed, low power consumption and adaptability of the human brain for certain functions, such as performing cognitive tasks. For devices to mimic the performance of the brain, multi-level storage and a high storage density are needed. We have demonstrated interface driven memristive effects, at room temperature, in Schottky interfaces between Nb-doped SrTiO3 (Nb:STO) and ferromagnetic metals, without the need of a forming process. Multi-level switching originates from well controlled and tailored interface effects, unlike bulk effects that drives conventional memristors. The Schottky interface of Nb:STO offers rich electronic properties that have been shown to be useful both for memristor and spintronics applications. Temporal dependence of these memristors were studied and used to mimic learning and forgetting in short-term memory in human brain. We are currently working with researchers from the Bernoulli Institute to develop phenomenological and mathematical models, derived from the outputs of our memristor training protocols. This will enable predictive memristive behaviour for large scale integration .
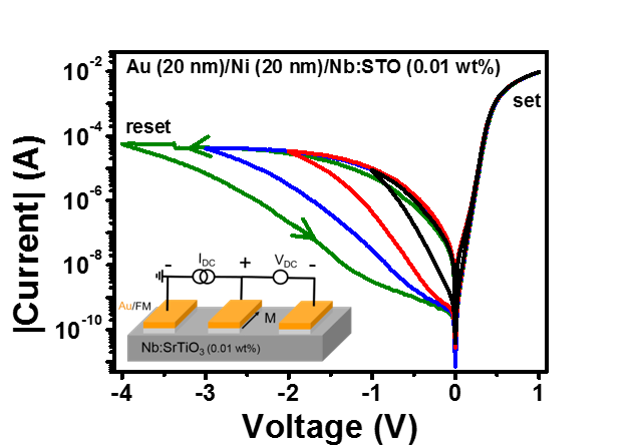
(5) Spin momentum locking and hot electron transport using topological insulators:
Topological insulators are intriguing in that they can host electrically conducting surface states along with an ideally insulating bulk. Bi2Se3 thin films for this work were grown at Rutgers University and were fabricated into nanodevices at NanoLab NL. The intrinsic locking of the electron’s spin to its momentum, leading to a dissipationless current flows was demonstrated using Co detectors in these thin films. Several cross checks were performed to ascertain factors beyond spin momentum locking that could influence the detected spin voltage. Using the High Field Magnet laboratory at Nijmegen, it was also established that besides the usual surface states, other conducting pathways are coupled to the bulk insulating states in such topological insulator films, not known earlier. The influence of metal contacts on topological insulators are also studied using a hot electron transistor scheme (PhD: Eric de Vries, Master students: Bart Zillen, Arjan Burema).
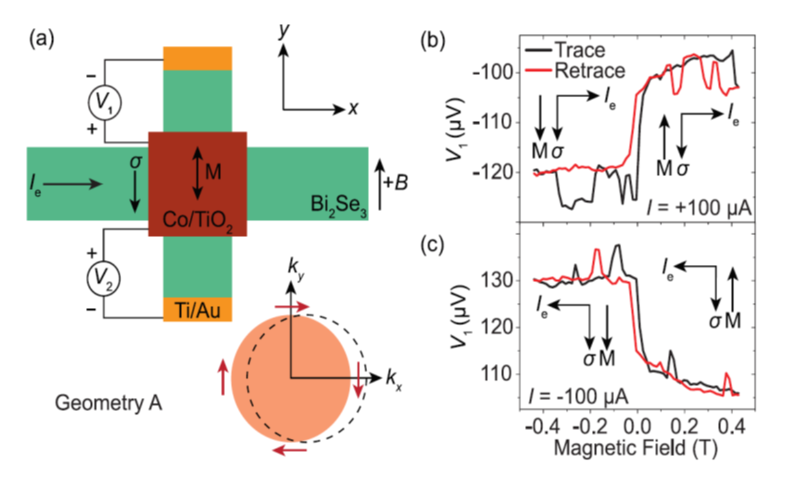
Last modified: | 10 September 2023 10.58 a.m. |