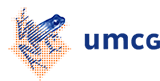
IRMS
Isotope ratio measurements
Stable isotope studies are performed in humans as adults or newborn infants and laboratory animal models such as rats and mice. In a number of situations 13C enrichment is measured in breath CO2. In the majority of cases compound specific analysis is needed in metabolites in plasma. The metabolites involved are present in plasma in a large range of concentrations. Glucose and cholesterol are at the level of 3 – 5 mmol/l or higher, triglyceride fatty acids at the level of the high umolar / low mmolar range, bile acids at the low umolar range, i.e. 1 – 4 umol/l in healthy adult subjects. Dynamic measurement normally involves repetitive sampling of blood in order to determine time dependence. The amount of available blood becomes limiting in newborn infants, rat and mice. This creates high demands on the analytical methodology. Downscaling of the detection limits is necessary. This can be approached by adjustments in the sample preparation procedure to obtain higher recovery rates and smaller end volumes or otherwise by choice of instrumentation. The other demand is created by the amount and type of label to be administered. In this respect it is of importance whether or not a loading test is necessary and whether or not a continuous infusion must be a applied. Also the type of labeled substrate plays an important role. Highly enriched, synthetic substrates are often expensive but can be administered in relatively low dosages. Substrates as natural corn starch products are only available at the low enrichment of about 0.02 at% per C-atom. In this case a large loading dose of the labeled product is required.
Regular analytical techniques used today for compound specific isotope analysis are continuous flow isotope ratio mass spectrometry (CF/IRMS) and gas chromatography mass spectrometry (GC/MS).
Continuous flow isotope ratio mass spectrometry
CF/IRMS is used in the stand alone mode for analysis of 13C-abundance in breath CO2 or for compound specific analysis in suitable metabolites by selective conversion to CO2 in the biological matrix. The latter is exemplified by the measurement of the 13C-abundance of plasma urea. For this purpose urea in plasma is converted to CO2 in an exetainer tube using urease enzyme. The head space is sampled and automatically analyzed with CF/IRMS. Normally, compound specific isotope analysis in biological matrices at low enrichment level is determined using gas chromatography (GC) interfaced to isotope ratio mass spectrometry using a reactor interface. In case of 13C abundance measurements organic compounds are combusted on-line to CO2 and water applying a capillary combustion furnace (GC/C/IRMS). Extension of the system with a reduction furnace, nitrogen containing compounds like amino acids can be subjected to 15N/14N isotope ratio measurements. Deuterium enrichment in metabolites can be determined using a pyrolyis interface (GC/Pyrolysis/IRMS) converting the metabolite to carbon monoxide and hydrogen. On-line transfer of produced hydrogen into the IRMS allows measurement of 2H/1H isotope ratio’s. The on-line techniques coupled to IRMS permits measurement of low enrichment levels, but require relatively large amount of metabolite in order to assure adequate accuracy. The demand for the required amount of metabolite is determined by the number of isotope atoms in the molecule and the natural isotope abundance of the heavy isotope. Carbon is prominently present in organic molecules and the 13C natural abundance is near 1.1 at%. This supports a sensitive method in terms of the required amount of material. Roughly spoken 10 ng organic compound is the lower limit for accurate 13C determination. Hydrogen is also prominently present but the natural abundance of 0.015 at% is very low. The minimally required amount of organic metabolite is around 100 ng. The natural abundance of 15N is intermediate (0.34 at%) but normal only one or maximally 2 nitrogen atoms are present in an organic molecules like amino acids. Therefore at least 100 ng organic substance is needed for accurate 15N measurements. GC/C/IRMS and GC/pyrolysis/IRMS normally require derivatization , which leads to isotope fractionation and dilution of the enrichment. A disadvantage of the technique is that the instrumentation must be adapted for the isotope to be measured by changing the reaction furnace. A new development is the recent introduction of commercial instrumentation for LC/C/IRMS enabling 13C isotope abundance measurements of metabolites after separation on high pressure liquid chromatography (HPLC). So far only combustion and 13C-analysis can be performed.
Gas chromatography / mass spectrometry
GC/MS has been used for decades to determine enrichments of stable isotopes at a relatively high enrichment level. Generally the enrichment level should exceed 0.5 mol% although positive exceptions have been documented in case multiple labeled substrates have been used such as 13C5 and 2H7-cholesterol for the determination of cholesterol absorption efficiency applying only 15 mg 13C5-cholesterol intravenously and 30 mg 2H7-cholesterol orally. The natural isotopomer abundances are very low in this application. Therefore enrichments down to 0.01 mol% could be measured accurately. GC/MS does not convert compounds to small gaseous molecules as does GC/reaction/IRMS. The derivatized metabolite is ionized in the ion source and fragmented to charged fragments with different masses. A suitable charged fragment is chosen and the isotopomer distribution of the fragment is measured. The distribution is dependent on the elemental composition of the fragment and consists of the intensity of mass M0, M1, M2, M3, etc. M0 represents the probability that all elements are present as the most abundant lightest species (12C, 1H, 16O, 14N). M1 is one mass unit higher, representing the situation that one higher isotope is present. The abundance of M1 is mainly determined by the contribution of 13C, being 1.1% per C-atom. A molecule containing 20 C-atoms will show a M1 contribution of about 22-23%. M2 represents the probability that 2 13C-atoms are present and its contribution is much lower than that of M1. M3, M4, M5 etc follow in decreasing abundances based on the probability that 3,4 or 5 13C atoms are present. The GC/MS approach has many advantages. The enrichment measurements are independent on the type of isotope. Derivatization does not reduce the level of enrichment in GC/MS although the baseline isotopomer abundance may be affected by derivatization as is the case in Silicon containing derivatives. Si has high abundant higher isotopes. The major advantage is that GC/MS is very sensitive in terms of required amount of metabolite. Picogram quantities are often sufficient for accurate measurement. Therefore the technique can be applied at low metabolite concentration levels and in small sample sizes. The sensitivity can even be manipulated by the choice of the type of derivatization and the choice of ionization technique (electron impact, positive chemical ionization applying different reagent gases, electron capture negative ionization. Multiple isotope labeling of the substrate does not increase the enrichment of the metabolite, but moves the measurement to a higher isotopomer with a lower natural abundance. This improves the signal to background ratio in enriched samples. The major disadvantage of GC/MS is the high lower limit of enrichment needed for accurate isotope enrichment measurements.
Infrared spectrometry for isotope abundance measurements (SIRIS)
Infrared spectrometry is gaining access to biomedical stable isotope applications. Sofar the most abundant use is in breath 13CO2 analysis in relation to clinical 13CO2 breath tests. Non Dispersive Infrared spectrometry (NDIR) is used more and more in the area of patient care introduced by the 13C-urea breath test for the detection of a Helicobacter Pylori infection in the stomach. The advantages over FF/IRMS are the low cost, simplicity of required infrastructure and use, the small size and therewith the potential to use it in the physicians office. The introduction of the technique into the clinical setting for the purpose of the 13C-urea breath test may stimulate the use of other clinical breath tests as described above. Besides NDIR, also laser infrared techniques have been developed and are being developed for breath 13CO2 analysis. So far the available NDIR instruments have shown their accuracy when applied to human breath and by using breath collection bags of 50 ml. This is a contrast to CF/IRMS which uses 10 ml exetainer tubes. The use of collection bags is due to the fact that NDIR is less sensitive and as such require lager volumes of breath for accurate measurements. This approach is very useful at a small scale, as is the case in the Helicobacter Pylori diagnosis when only 2 samples are to be measured for each patient. In research settings storage of bags become problematic when large groups of patients or volunteers are tested and 20 or more samples are to be collected per patient. Newer developments in NDIR focus on the use of breath collection tubes as used with IRMS. However, developments are focused on the sample introduction only, not on enhancement of sensitivity of the detection system itself. The demand on increased sensitivity is originating from pediatric departments when breath tests are to be performed in young children but also from research areas outside the biomedical field, such as the research related to atmospheric CO2 at high altitudes where CO2 concentrations are low and the air is very thin. Laser infrared spectrometry developments also focus on the measurement of deuterium and 18O abundance in water. Commercialized systems in the future may become very useful in the clinical situation measuring energy expenditure and body composition. In the ideal situation future instruments may be equipped with various lasers allowing analysis of 13C in breath CO2 and deuterium and 18O in breath water vapor. As described above most of the biomedical research focus on compound specific isotope analysis using GC/MS in case of high enrichment measurements at low metabolite concentrations or in small samples or GC/reaction/IRMS at low enrichment levels in case sufficiently high metabolite concentrations or sufficiently large samples are available. In principle infrared technology may replace IRMS when a number of criteria are met. In the first place interfacing with existing reaction furnaces must be made possible and computer control must be established. So far reaction interface furnaces have been developed and patented by IRMS companies. It may therefore be necessary to develop specific reaction furnaces. Secondly the sensitivity of the infrared systems must be increased to meet the sensitivity of IRMS in terms of the minimal amount of CO2, H2, N2 needed for accurate measurements. This may require new designs in measurement cells and detection systems. Thirdly the infrared system must be able to work in a continuous flow mode dealing with carrier gas from the gas chromatograph and the interface system has to eliminate solvent from the HPLC. It has been mentioned already that a large disadvantage of GC/reaction/IRMS is the fact that measurements are isotope specific. Different reaction furnaces are needed for the different isotopes. This will not change when IRMS is replaced by infrared spectrometry. At most the instrument may become less expensive. It would be very beneficial when laser infrared technology can be used to detect isotope specific signals in organic molecules in a continuous flow mode. This would eliminate many disadvantages encountered in compound specific IRMS technology today.
Last modified: | 22 November 2012 4.09 p.m. |