The evolution of spatial pattern formation
When searching for food, shelter and other resources animals face the daunting complexity of the natural world, where resource distributions are often quite unpredictable. To cope with the challenge to maximize the probability of resource encounters, many organisms adopt specialized search strategies. Brownian and Lévy walks are prominent examples of random walk strategies where both the direction and step length of the constituent moves are drawn from a probability distribution. In case of Lévy walks, step lengths follow a power-law distribution (with a characteristic exponent μ), where many short steps are occasionally alternated with a long step. Several theoretical studies suggest that a Lévy walk with exponent μ ≈ 2 is the most efficient random search strategy in resource-limited environments where food occurs patchily at locations unknown to the searcher. Although shown to be optimal for only these specific conditions, Lévy walks with μ ≈ 2 are broadly found in nature, suggesting that they are actually adaptive over a wider range of conditions. In a recent study (De Jager et al., Science, in press), we hypothesize that this wide occurrence is due to the fact that resource distributions are often not completely determined by external factors, but also by the organisms consuming or otherwise using these resources. This feedback between resource and organism distribution leads to an intriguing interplay between ecology and evolution: natural selection should lead to movement strategies that an optimally adapted to the patterning of the environment; but the environment in turn is shaped by the movement strategies of the organisms.
We illustrate these general considerations by a concrete example that is amenable to experimental investigation. On intertidal flats, the distribution of regularly-spaced clumps of mussels (Mytilus edulis) results from the interaction between local mussel density and the crawling movement of young mussels. Pattern formation in mussel beds is attributable to two opposing mechanisms: cooperation and competition. Through movement into cooperative aggregations, mussels increase their local density, thereby decreasing wave stress and predation risk. Conversely, competition for algae, which occurs on a larger spatial scale than facilitation, prevents the formation of larger clumps by limiting the number of mussels within a long range. The interaction of local facilitation and long-range competition results in the emergence of a patchy distribution of individuals, which simultaneously reduces risk and minimizes competition for algae. The distribution patterns found in mussel beds are readily reproduced in lab experiments (Figure 1C). Investigating the movement patterns of individual mussels shows that – also in this system – the search strategies of mussels corresponds to a Lévy walk with exponent μ ≈ 2 (μ = 2.1 provides the best fit; Figure 1AB). Individual-based simulations confirm that such a search strategy does indeed result in the spatial patterns characteristic for mussel beds (Figure 1D).
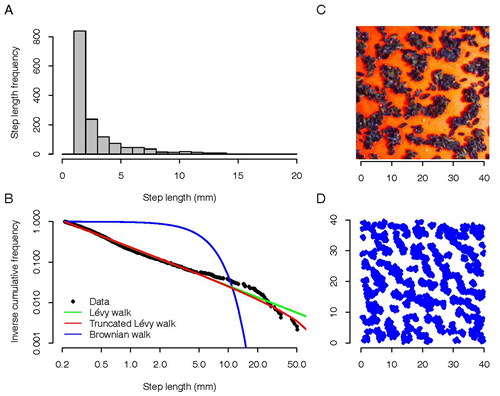
An evolutionary analysis based on adaptive dynamics methods revealed why a Lévy walk strategy with μ = 2.1 is superior to other movement strategies. To this end, we considered a wide spectrum of movement strategies. For each strategy m, we considered the 'resident' population consisting of individuals all exhibiting strategy m. For each such strategy, the individual movements will result in a characteristic pattern P(m) (= a characteristic spatial distribution of mussels). Given this pattern, we could then calculate the reproductive success ('fitness') of all alternative strategies m'. Here we assumed that reproductive success is dependent on the energy spent moving and the energy gained by food consumption (reflecting local competition with other mussels). The resulting fitness function F(m',m) could be analyzed by the methods of adaptive dynamics. It turned out that the only evolutionarily stable and convergence stable strategy was a Lévy walk with exponent μ = 2.1, as found in our experiments. Interestingly, this exponent is larger than the value μ = 2.0, which leads to a spatial pattern of mussels that is optimal in the sense that it maximizes the average fitness of the population. Hence, evolution does not optimize the ‘performance’ of a mussel bed as a whole. From an evolutionary perspective, this is not surprising, since natural selection should maximize individual fitness rather than population fitness. The spatial pattern of a mussel bed may be viewed as a 'public good'. A mussel bed generated by the resident strategy μ = 2.0 is ‘vulnerable’ in the sense that the resident strategy can be 'exploited' by mussels with a strategy μ > 2.0. Only the (ecologically sub-optimal) strategy μ = 2.1 cannot be exploited by alternative strategies and is, hence, evolutionary stable.
This is one of the first examples demonstrating how large-scale spatial patterns may result from the interplay of ecology and evolution.
Last modified: | 03 December 2015 12.54 p.m. |