The interplay between genetic and environmental stress
Research of the Conservation & Population Genetics section is focused on various population genetic topics and includes projects that centre on unravelling the genetic basis of ageing in Drosophila and on modelling the ecological implications of the spread of genes from transgenic crop into wild relatives. Most emphasis, however, is directed towards understanding the dynamics of genetic variation in small, endangered populations (conservation genetics). We approach these questions both theoretically and experimentally, the latter by using Drosophila melanogaster as model organism. The experimental projects mainly centre on fundamental ../../index to gain an insight into the genetic processes that underlie genetic erosion, inbreeding depression, population extinction, and the role of gene flow in relation to these processes. In recent years we have particularly focused on the interaction between genetic stress (inbreeding and inbreeding depression) and environmental stress (like thermal stress). Some of the results in this ../../index area are outlined in more detail below. These presented data are mainly the result of the PhD projects by Desirée Joubert and Joke Bakker. The latter project was co-supervised by Franjo Weissing.
Human impact on nature has increased extinction rates of populations and species dramatically. Many plant and animal species currently have to cope with human induced alterations of their natural environment. Climate is changing globally at a speed that is higher than observed in the past 10,000 years and projections into the future show an acceleration of global temperature rise. Chemical pollution and other alterations in the physical habitat do cause environments to become more and more hostile. At the same time changes in species distributions and the introduction of exotic species will change mutual relationships causing e.g. competitive increased competition or the spread of novel diseases. To meet these anthropogenic changes of the environment it is essential that populations can cope with the imposed stresses at both the short-term and the long-term. At the short-term the initial tolerance and the adaptive plastic response (phenotypic plasticity) are decisive parameters. At the more long-term evolutionary adaptive responses are of paramount importance. As we are mostly dealing with short or intermediate time scales, beneficial mutations will be rare and consequently the evolutionary response initially will mainly depend on the standing genetic variation. Hence, the ability to cope and adapt to changing conditions will depend both on how well individuals can adjust to the new conditions and on the amount of genetic variation for relevant fitness traits available for evolutionary responses present in a population.
Unfortunately, on top of above mentioned environmental changes, human induced destruction of habitats has caused once large populations of many species to become small and fragmented. Subdivision of once large populations into small population fragments (subpopulations) in combination with limited gene flow between fragments, because the distance between suitable patches increases (metapopulation structure), will affect the availability of genetic variation greatly. From a population genetics perspective, small, isolated populations are subject to genetic drift, resulting in both loss of (adaptive) genetic variation and an increase in inbreeding coefficient. The latter is most often accompanied by inbreeding depression affecting most fitness components negatively. This has severe consequences for the adaptive response of such populations to environmental changes (stress) as the consequences of genetic erosion are expected to impede both the tolerance to stress and the evolutionary potential to adapt to stresses.
To investigate these consequences of genetic erosion for adaptive responses we assessed the effect of inbreeding on fitness (measured as egg-to-adult survival) in relation to temperature stress. To this end, we compared the egg-to-adult survival (percentage of eggs the become adults) of inbred and non-inbred populations at two near optimal temperatures (21°C and 25°C) and at two more extreme (stressful) temperatures (17°C and 29°C). Figure 15A shows that inbred populations show on average a significantly decreased egg-to-adult survival. The variation in egg-to-adult survival among inbred populations is large, which is typical for inbreeding as the amount of deleterious variation fixed in a population is governed by genetic drift. This decrease in egg-to-adult survival is observed at all temperatures. Consequently inbred populations show already a decreased fitness and lower tolerance even under near optimal environmental conditions. The difference in mean egg-to-adult survival among populations shown in Figure 15A may obscure the changes in egg-to-adult survival in relation to temperature. Therefore, we standardize egg-to-adult survival (calculated as the egg-to-adult survival at each temperature relative to the highest egg-to-adult survival viability observed for that population at any temperature). Figure 15B shows that egg-to-adult survival decreases towards the more extreme temperatures. Most importantly, however, this decrease in fitness at the extreme temperatures is significantly larger for inbreds than for non-inbreds, although this again varies considerably among populations. This shows that inbreeding not only compromises fitness (egg-to-adult survival) but that this effect becomes enhanced under stress conditions. A similar effect was also observed for a number of morphological characters in this experiment (data not shown). There is increasing evidence that in general, although there are exceptions, environmental stress does increase inbreeding depression. In conclusion, whereas adaptive plasticity is crucial for a population to cope with the imposed stress at the short term, one aspect of genetic erosion is that such responses can be significantly impaired by inbreeding.
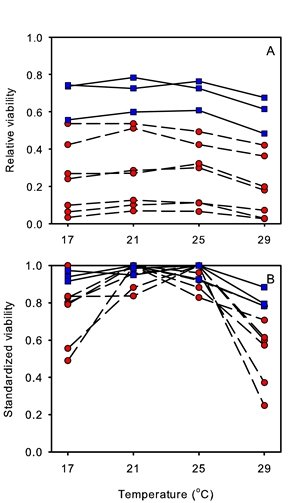
A second aspect of genetic erosion in fragmented populations is that small isolated populations undergo increased levels of genetic drift. This causes genetic variation originally present within a large population to become redistributed among the remaining small populations. As different alleles will get fixed in different small populations, this will result in substantial genetic differentiation among the populations, where some may lack (fixed for the non-adaptive variants) or have only low frequencies of the adaptive variants while others are (nearly) fixed for the adaptive variants. This would not only affect the tolerance to stress, but also the evolutionary potential of small population as less (adaptive variation is available.
To test this possibility, we used metapopulations that had been fragmented for 40 generations in an experimental setup as shown in Figure 16. After the 40 generations of fragmentation, the initial tolerance to each of these small populations for different stresses was assessed, measured as egg-to-adult survival. Figure 17 (left panel, before adaptation) shows the initial tolerance to high temperature stress of six metapopulations (M1-M6). Before adaptation there is substantial variation among subpopulations within metapopulations (open circles for each bar) with respect to tolerance to high temperature stress as a consequence of genetic drift in the subpopulations. This variation turned out to be due to one or a few recessive deleterious alleles that, when homozygous, caused near lethality at high temperature (29°C) but does not affect fitness at benign temperature (25°C). As all populations were started from the same base population this variation in tolerance reflects the variation in allele frequencies due to genetic drift. Clearly, fragmentation can lead to significant variation in tolerance, some subpopulation showing maximal fitness while others have a tolerance near zero.
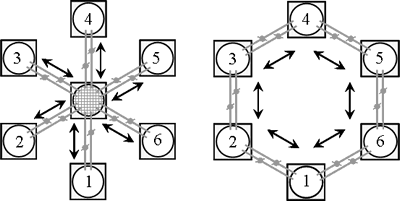
To investigate how subdivision affects the adaptive potential of fragmented populations, the subpopulations individually were adapted to high temperature (28-28.5oC) and the tolerance was thereafter tested again. The results are shown in Figure 17 (left panel, after adaptation). After adaptation the distribution in viability has become highly bimodal: many subpopulations have adapted and show a near optimal viability under stress, but a subset show a near zero viability. As a consequence of subdivision, the latter subpopulations seem to have lost the adaptive allele(s) and become fixed for the sensitive allele. These are unable to adapt and are expected to go extinct under high temperature stress.
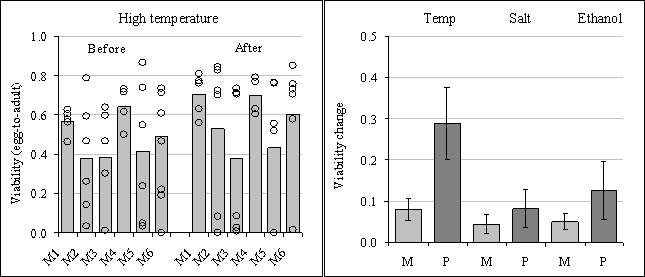
At the same time we compared the adaptive potential (the change in egg-to-adult survival) of the metapopulations with six undivided populations of near the same size (around 250 individuals) of the total metapopulation size. This was done for three different stresses (high temperature, salt stress and ethanol stress). The results show that metapopulations on average have a smaller adaptive response (improve in egg-to adult survival) than undivided populations for the three stresses, particularly so for high temperature stress (Figure 17, right panel). This is due to the fact that a number of subpopulations have become fixed for the high temperature sensitive allele(s) and cannot adapt (Figure 17, left panel). One has to realize however, that the variances are pretty large, which is to be expected after a long history of genetic drift. In short, the metapopulation experiment shows that compared to large undivided populations, fragmented populations will show lower levels of standing genetic variation and, consequently, their adaptive potential is necessarily decreased. Clearly, both initial tolerance and the evolutionary adaptive response will become impeded after a history of fragmentation.
Summarizing, both experiment show that fragmentation of (formerly) large populations due to ongoing habitat destruction induces genetic processes that necessarily do limit adaptive responses to changing environments, both at the short-term and the long-term. This implies that ongoing fragmentation can significantly enhance the adverse effects of human induced alterations of the environment (like climate change) and does endanger the persistence of biodiversity significantly. Promoting sufficient gene flow between fragments will therefore be an important management measure, as this will, at least partly, restore the adaptive potential. Moreover models built to predict the persistence of biodiversity under anthropogenic stress should preferably take the genetic consequences of population fragmentation into account.
Last modified: | 03 December 2015 12.54 p.m. |