Evolution of self-organized division of labour
The phenomenon of division of labour is found throughout all levels of biological organization. We can define division of labour as the collaboration of different individuals regarding the performance of certain tasks, where different individuals (or sub-groups of individuals) perform consistently different tasks (or set of tasks). The different tasks available are therefore performed simultaneously. The successful performance of all tasks allows for a common goal to be reached. The concept of division of labour was first enunciated regarding human societies. In nature, examples of division of labour exist in some species with bi-parental care, such as hornbills and house sparrows, where males and females have different roles in raising the offspring. In group-hunting mammals, such as lions, killer whales, dolphins and chimpanzees, different individuals fulfill specific tasks: some chase the prey, others surround it, and others kill it. Eusocial insects, such as ants, bees, wasps and termites, are perhaps the most extreme example of non-human division of labour. Different tasks, such as brood care, queen tending, nest maintenance, and foraging are performed by specialized groups of sterile workers. Various factors (genetic, environmental and developmental) seem to influence which tasks workers are more likely to perform at a given moment.
Workers of an insect colony do not have a general overview of the state of the colony, nor does a central command exist that distributes orders on which tasks to perform. It has been suggested that workers must make choices based on local cues of different behavioural stimuli and information obtained from nest mates. From the interaction of individual choices, a pattern of division of labour emerges in a self-organized manner. Several self-organization models have explored the type of behavioural rules that can lead to division of labour in social insects. These models are successful in replicating patterns of division of labour that are observed in nature, such as specialization and age polyethism (different tasks being performed by individuals of different age cohorts), but the analysis of such models typically stops once the desired pattern is reached. However, due to the impact of division of labour on colony fitness, it is expected that the rules of individual behavior underlying division of labour are targeted by natural selection to produce adequate colony-level response. One does not expect emergence of division of labour to be maintained if it is disadvantageous to colonies. The role of natural selection in shaping behavioural rules is completely overlooked in current self-organization models. On the other hand, models that explicitly analyze the adaptive value of division of labour, totally neglect the behavioural mechanisms behind it. Ideally, one would develop theory that integrates self-organization and evolutionary perspectives, but what is indeed most often found is that they are studied separately. The need to explore the interaction between self-organized processes and evolution in the study of division of labour has been recognized before, but little has been done to accommodate it.
We developed models that integrate a self-organizational and evolutionary perspective. Characteristically such models must consider an ecological and evolutionary timescale. On the ecological timescale, individuals interact and perform tasks, therefore self-organization occurs. On the evolutionary timescale, the behavioral rules that will allow the emergence of division of labour evolve. A general scheme for such models is depicted in Fig 1. As a starting point we used already existing self-organization models, to which we added evolution, by considering a simple genetic architecture for the behavioral rules individuals obey. Our main interest was to analyze to what extent the behavioral rules chosen in these self-organization models hold when evolution is incorporated in the models.
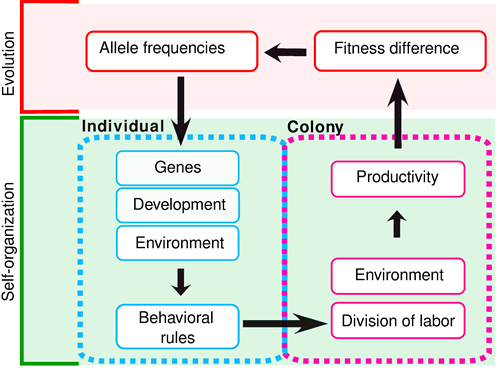
We began by looking at the so-called fixed response threshold model, one of the standard models for self-organized division of labour. According to this model, individuals in a eusocial insect colony have internal response thresholds for task-associated stimuli. Furthermore, the model assumes the presence of task-specific stimuli which have the tendency to increase in time but which are reduced when individuals are working on the task. An individual will perform a task if the stimulus for this task exceeds the threshold value. It is a classical result of the self-organisation literature that the fixed-threshold model can produce specialization if the individuals in a colony differ substantially in their thresholds (Fig 2).
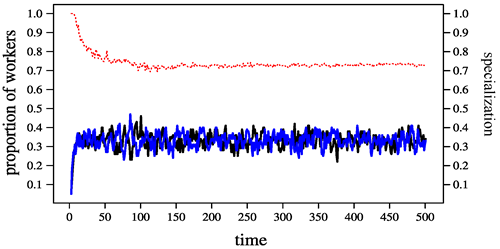
We allowed for the evolution of thresholds to test whether specialization would evolve from a homogeneous population. Colony fitness was calculated on the basis of colony performance (the number of acts performed for each task at the end of a generation). Colonies produced sexual individuals in proportion to their fitness and these new individuals mated and founded new colonies. It turned out that this straightforward implementation of evolution did not result in colonies with division of labour. To achieve this, an incentive for task specialization had to be included in the model.
Two reasons are commonly given as adaptive advantages of specialization. One is the possible increased efficiency of specialized workers due to their experience with a given task. The other are the costs involved in switching from one task to the other. When adding such switching costs in our model, the thresholds evolved to a bimodal distribution allowing specialization (Fig 3). This is one of the first models where self-organized division of labour evolved from scratch.
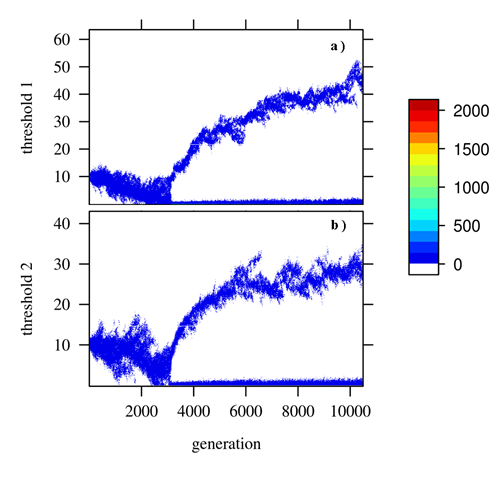
We observe that for individuals to specialize there must enough individuals with high thresholds for the first task and low thresholds for the second task and enough individuals with low thresholds for the first task and high thresholds for the second task. The stimulus for a given task should be kept in check by those workers with the lowest thresholds for the task. Otherwise, the stimulus increase will cause other workers, with higher thresholds, to have the same probability to engage in the task.
Due to recombination and random mating, only a part of the colonies will have an appropriate combination of individuals to produce specialization (Fig 4). Overall we observe a negative correlation between thresholds evolving, such that if an individual has a high threshold for a task, it will have a low threshold for the other task (Fig 5).
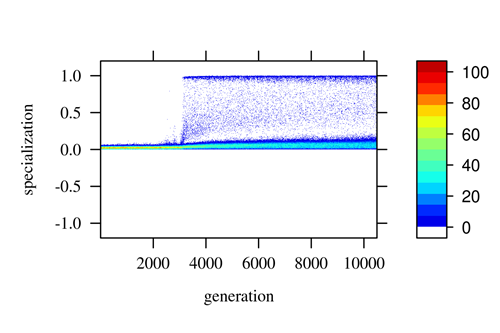
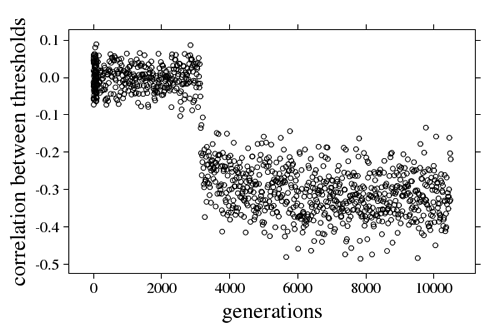
The main advantage from including an evolutionary perspective in a self-organization model of division of labour is that it allows us to think about what traits should evolve to cause division of labour and why. Our first model is highly simplistic and of a conceptual nature, but already shows that the evolution of division of labour is not a trivial matter. Further research will focus on more mechanistic models for division of labour, where the link between fitness and task allocation is approached more realistically.
Last modified: | 03 December 2015 12.54 p.m. |