The effect of temperature on sex determination - On the co-evolutionary dynamics of environmental and genetic sex determination
In many cold blooded species, like many reptiles and fish, the sex of an individual is determined by temperature during embryogenesis with some temperatures leading to female and others to male development. This is called temperature dependent sex determination (TSD). In contrast, in most other organisms, such as all mammals and birds, the sex of an individual is determined by its genotype. Such a system is called genetic sex determination (GSD). Recent work on the molecular basis of sex determination has revealed that the dichotomy between GSD and TSD may not be as sharp as previously believed. The underlying gene networks are quite similar and small changes can convert a GSD system into TSD and vice versa. In addition, more and more species are being discovered where both genes and temperature affect sexual development, and where multiple sex determining (SD) systems coexist within one species. Phylogenetic analyses strongly indicate that multiple transitions between GSD and TSD, in both directions, have occurred.
There are several hypotheses regarding the evolution of TSD from GSD, but by far the most influential hypothesis is the so called Charnov & Bull hypothesis. The basic idea is that the fitness of males and females is differentially affected by variation in environmental conditions. Specifically, under some conditions females have higher expected fitness than males, while other conditions benefit males more. TSD is a mechanism that allows for flexible adjustment of sex ratios in situations where biased sex ratios are selectively favoured. GSD does not readily allow for such flexible facultative sex ratio adjustments, thus giving TSD a selective advantage in these cases. However, the very flexibility of TSD can also be a liability when fluctuations in temperature between years are sufficiently large. In that case, years with extreme temperatures may lead to very biased sex ratios among the offspring, and thereby cause (near) population extinction. This is less of a problem in long-lived species, where the fluctuations over a lifetime tend to cancel each other out.
Here we present a model that is not based on the assumption of environment-dependent sex-specific fitness effects, but is based on selection for specifically biased sex ratios due to local kin competition. Sex ratio theory predicts that the more dispersive sex should be overproduced since it is least likely to compete with relatives for resources or space. The basic idea is that under these conditions TSD is likely to evolve because it allows for specifically biased sex ratios and therefore has an advantage over GSD. In the model the degree of environmental fluctuations and longevities was systematically varied to understand the interplay and importance of the different factors. Starting with a GSD population it was investigated whether and under which conditions TSD might evolve.
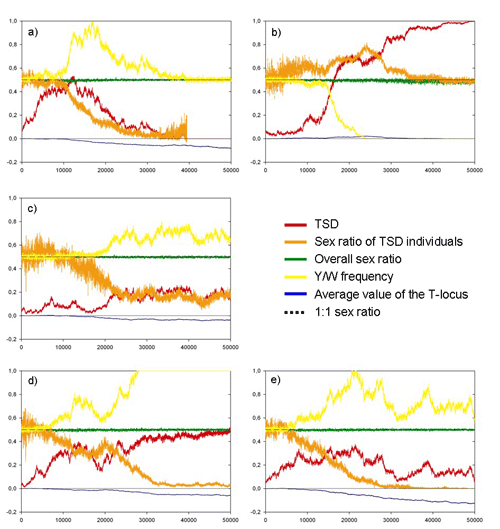

Five different outcomes were observed (Fig. 1): (i) the initial GSD state was stable; (ii) TSD evolved and took over; (iii) a new type of GSD evolved at a second locus; (iv) GSD and TSD stably coexisted; (v) multiple coexisting GSD systems evolved. In line with earlier work, TSD evolved more easily when environmental fluctuations were small and when lifespan was long (Fig. 2).
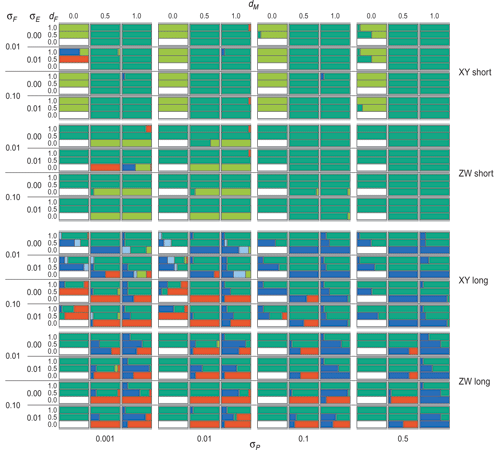

In the simulations rapid evolutionary transitions between GSD and TSD were common even without the assumption of temperature dependent sex-specific differential fitness, thus offering a new, additional explanation for the evolution of TSD. Another important insight from the model is that multiple sex determining systems can evolve from identical initial conditions, which may be governed by random factors such as genetic drift.
Last modified: | 03 December 2015 12.54 p.m. |